CHAPTER 5. CLOSED-COURSE STUDY
Pedestrian crossing traffic control options include numerous combinations of signs and flashing beacons/LEDs. The breadth of combinations gives rise to a number of design variables; the optimal combination of these variables that results in long-term driver yielding to pedestrians (rather than just a “honeymoon phase” of compliance) has not been established. Several design factors have not been fully researched to determine the optimal design, including the following:
- Flash rate of beacons or LEDs.
- Shape and size of beacons/LEDs (round, rectangular, 8-inch, 12-inch, 5- by 2-inch, etc.).
- Placement of beacons or LEDs in the assembly units (within the sign, top, sides, bottom, overhead, in-road, etc.)
- Placement and message of signs and markings (warning and/or regulatory) for uncontrolled crossings (with and without beacons/LEDs).
- Brightness and how it influences driver behavior and how it affects glare and message recognition (especially given various lighting conditions such as day, night, fog, rain, snow).
- Influence of vehicle speed.
This research endeavors to provide the analytical basis for addressing several of these variables. Although it will not address every variable, the findings build a foundation and future research can fill the remaining gaps.
There are several questions regarding the optimal configuration of beacons used with Pedestrian Crossing signs (abbreviated as “Ped X-ing” in tables and figures in this document). Specific research objectives for this closed-course study are the following:
- Determine whether the shape, size, and placement of flashing beacon/LEDs affects the following:
- Sign legibility distances (legibility distance is the location upstream of a sign where a driver can correctly read all of the words on a sign or correctly identify the symbol on a symbol sign).
- Object detection.
- Sign legibility distances (legibility distance is the location upstream of a sign where a driver can correctly read all of the words on a sign or correctly identify the symbol on a symbol sign).
- Determine driver ratings of disability glare for 8-inch circular beacons and LED-embedded signs using a rapid flash pattern.
- Identify up to two assemblies for field evaluation to be conducted following the conclusion of the closed-course tasks.
As part of this FHWA study, participants drove a Texas A&M Transportation Institute (TTI) vehicle (a 2009 Ford Explorer) on a closed course at the Texas A&M University Riverside Campus. During each lap, the participants viewed 8 study assemblies, 9 distractor signs, and up to 11 objects. Each participant drove the course twice, with a pause between laps for the field crew to switch the signs and objects for the second lap. Lessons learned from a previous study demonstrated that the position of traffic control devices on the course could affect the detection distance.(25) Therefore, researchers rotated the assemblies during this study. The rotation was done when approximately 25, 50, and 75 percent of the participants had completed the study. Figure 21 shows the driving study course, along with the positions of the signs and objects (when present). Based on the available budget, the goal was to have 64participants in the study; therefore, researchers planned rotations to occur after 16 participants drove the course.
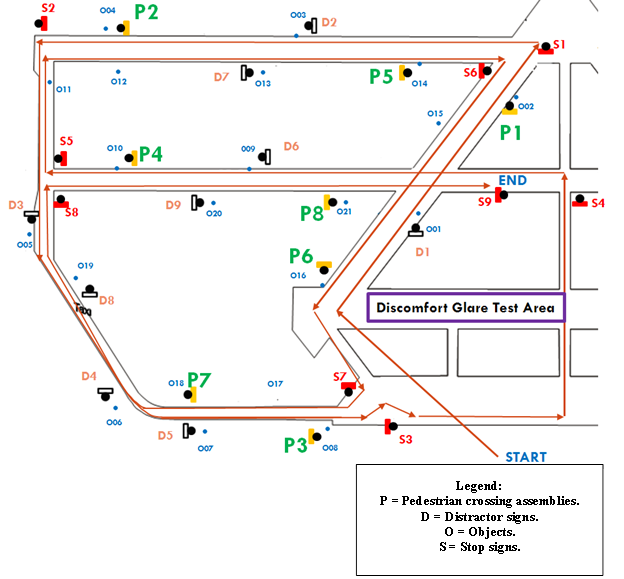
Figure 21. Diagram. Test route for Riverside study along with signs and objects positions.
The tasks for the participants while driving the route were to indicate when they could first do the following:
- See warning lights.
- See road signs.
- Read the words or identify the symbol on the road signs.
- See objects (pedestrian, trash can, or box).
As soon as the driver said “lights,” “sign,” “object,” or read the words/numbers/symbol on a road sign, the experimenter pressed a key on the laptop computer, which placed a mark in the file to indicate detection. Associated with this mark would be the Global Positioning System (GPS) coordinates of the vehicle along with the vehicle’s speed and the time the key was pressed.
After participants completed the driving portion of the study, researchers directed them to the discomfort glare portion of the study. The driver would position the vehicle at set distances from an assembly and would then answer questions following increases in the brightness of the beacons or LEDs. Figure 22 is a plan view of the discomfort glare study layout.
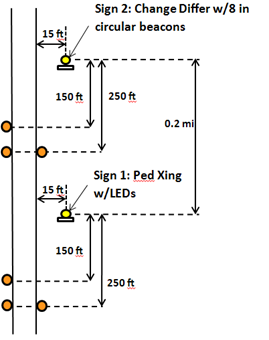
Figure 22. Diagram. Layout for the discomfort glare study.
The runway system on Texas A&M Riverside campus served as the test roadway for data collection. The runways offer a mixture of long straightaways, short intersecting segments, and curves. Researchers designed a route to permit participants to see every study assembly at a distance of at least 2,100 ft. To maximize the number of study assemblies, participants drove each runway section in both directions during a single lap. Each subject drove two laps, between which research team members changed the face of all distractor and study assembly signs, and the location of the objects (gray boxes, trash cans, and pedestrians).
Researchers added the following devices and objects to the Riverside runways:
- Pedestrian crossing assemblies that included a sign with or without optional beacons.
- Stop signs and distractor signs.
- Objects.
Pedestrian Crossing Assemblies
The study assemblies selected included the following:
- Two circular 12-inch beacons located above the sign (named C-A12 in the study) (see figure 23 and figure 24).
- Two circular 12-inch beacons located below the sign (C-B12) (see figure 25 and figure 26).
- Two circular 8-inch beacons located below the sign (C-B8) (see figure 27 and
figure 28). - One circular 12-inch beacon located above the sign and one circular 12-inch beacon located below the sign (C-V12) (see figure 29 and figure 30).
- Two rectangular beacons located above the sign (R-A) (see figure 31 and figure 32).
- Two rectangular beacons located below the sign (R-B), the format currently being used for the RRFB device (see figure 33 and figure 34).
- Sign with LEDs embedded into the border (LED) (see figure 35 and figure 36).
- Diamond-shaped sign with no beacons or LEDs (WO-B) (see figure 37 andfigure 38).
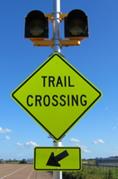
Figure 23. Photo. C-A12, lap A study assembly.
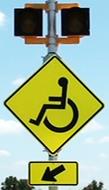
Figure 24. Photo. C-A12, lap B study assembly.
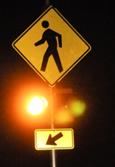
Figure 25. Photo. C-B12, lap A study assembly.
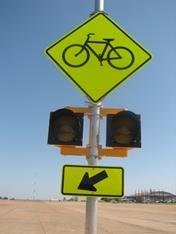
Figure 26. Photo. C-B12, lap B study assembly.
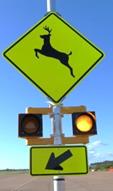
Figure 27. Photo. C-B8, lap A study assembly.
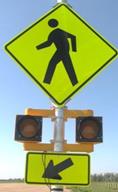
Figure 28. Photo. C-B8, lap B study assembly.
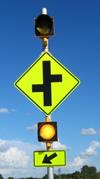
Figure 29. Photo. C-V12, lap A study assembly.
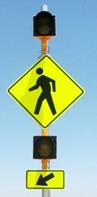
Figure 30. Photo. C-V12, lap B study assembly.
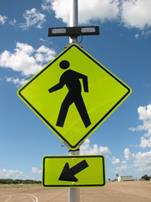
Figure 31. Photo. R-A, lap A study assembly.
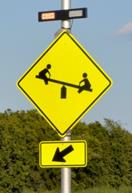
Figure 32. Photo. R-A, lap B study assembly.
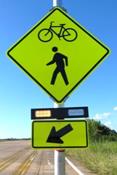
Figure 33. Photo. R-B, lap A study assembly.
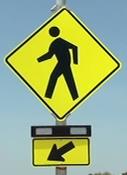
Figure 34. Photo. R-B, lap B study assembly.
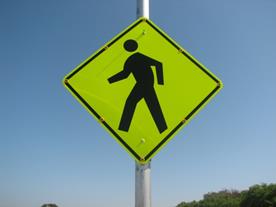
Figure 35. Photo. LED, lap A study assembly.
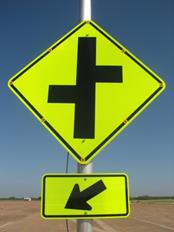
Figure 36. Photo. LED, lap B study assembly.
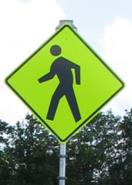
Figure 37. Photo. WO-B, lap A study assembly.
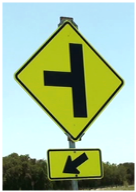
Figure 38. Photo. WO-B, lap B study assembly.
To serve as a baseline, one of the assemblies had neither beacons nor embedded LEDs. A mix of pedestrian crossing and other warning signs were selected for the study assemblies. The warning signs either had a pedestrian-related message or had a logo that was similar to a pedestrian. Study signs included Pedestrian Crossing (W11-2), Bike (W11-1), Playground (W15-1), Wheelchair (W11-9), TRAIL CROSSING (W11-15a), Pedestrian and Bike (W11-15), Deer (W11-3), T-intersection Ahead (W2-2), and Offset Intersections (W2-7L). All signs used in the assemblies had fluorescent yellow-green background so that the color of the signs used with beacons or LEDs would be similar. Table 93 describes the signs used along with the average retroreflectivity reading for each sign.
Table 93. Study assemblies used in closed-course Riverside track study.
Portiona |
Sign faceb |
Beacc |
Lapd |
Positione |
Retrof |
Driving |
Trail Crossing |
C-A12 |
A |
P4(I), P5(II), P6(III), P8(IV) |
783 |
Wheelchair |
C-A12 |
B |
P4(I), P5(II), P6(III), P8(IV) |
750 | |
Ped Crossing |
C-B12 |
A |
P2(I), P3(II), P4(III), P5(IV) |
906 | |
Bike |
C-B12 |
B |
P2(I), P3(II), P4(III), P5(IV) |
783 | |
Deer |
C-B8 |
A |
P8(I), P2(II), P3(III), P4(IV) |
703 | |
Ped Crossing |
C-B8 |
B |
P8(I), P2(II), P3(III), P4(IV) |
904 | |
Offset Intersection |
C-V12 |
A |
P6(I), P8(II), P2(III), P3(IV) |
812 | |
Ped Crossing |
C-V12 |
B |
P6(I), P8(II), P2(III), P3(IV) |
907 | |
Ped Crossing |
LED |
A |
P1 |
797 | |
Offset Intersection |
LED |
B |
P1 |
798 | |
Ped Crossing |
R-A |
A |
P3(I), P4(II), P5(III), P6(IV) |
879 | |
Playground |
R-A |
B |
P3(I), P4(II), P5(III), P6(IV) |
779 | |
Bike & Ped |
R-B |
A |
P5(I), P6(II), P8(III), P2(IV) |
724 | |
Ped Crossing |
R-B |
B |
P5(I), P6(II), P8(III), P2(IV) |
884 | |
Ped Crossing |
WO-B |
A |
P7 |
896 | |
T-Intersection Ahead |
WO-B |
B |
P7 |
911 | |
Comfort |
Change Differ |
C-B8 |
Comfort |
Discomfort Glare |
502 |
Ped Crossing |
LED |
Comfort |
Discomfort Glare |
691 |
aPortion = part of the study, either driving (for those assemblies viewed during the driving portion) or comfort (for those assemblies viewed during the discomfort glare portion). All study signs used on the driving portion of the study (i.e., lap A and lap B) were 36-inch, fluorescent yellow-green background color on type IV sheeting.
bSign Face = description of the face of the sign. Figure 23 to figure 38 show photographs of the sign faces and the types of beacons or LEDs included with the signs.
cBeac = type of beacons or LEDs included in the assembly: C = circular, R = rectangular, A = above, B = beneath, V = both above and beneath, 12 = 12-inch circular beacon, 8 = 8-inch circular beacon, LED = embedded LEDs on the edge of the sign, WO-B = without beacons (i.e., no lights associated with assembly).
dLap = assembly included in either lap A or B of the driving portion or as part of the discomfort glare potion.
ePosition = location of the assembly on the course depending on the rotation. The first number (P1 to P8) indicates position while the value within the parentheses (I, II, III, IV) indicates the rotation. Four rotations of the signs occurred during the study (see figure 21).
fRetro = average of four retroreflectivity readings in cd/lx/m2.
Assemblies Selected for Discomfort Glare Study
For the discomfort glare study, researchers asked the participants to park the study vehicle 250 ft and 150 ft away from a traffic sign assembly (see figure 22). The two assemblies tested included two 8-inch circular beacons mounted below a sign that said “Change Differ” (see figure 39) and a pedestrian crossing sign with embedded LEDs (see figure 40). The Pedestrian Crossing sign was 36 inches, fluorescent yellow-green background color on type 4 sheeting. The “Change Differ” sign was 36 inches with an orange background. A plan view of the study area is shown in figure 22, and an image from the start of the study area is shown in figure 41.
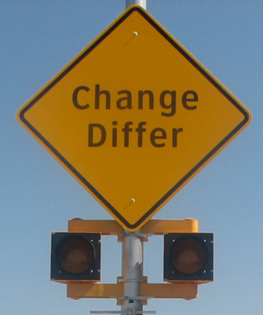
Figure 39. Photo. Discomfort glare assembly with circular beacons.
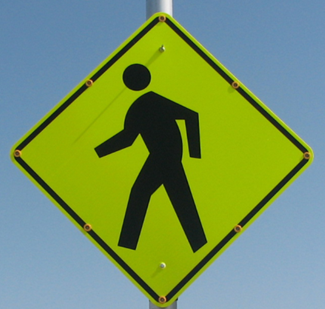
Figure 40. Photo. Discomfort glare assembly with embedded LEDs.
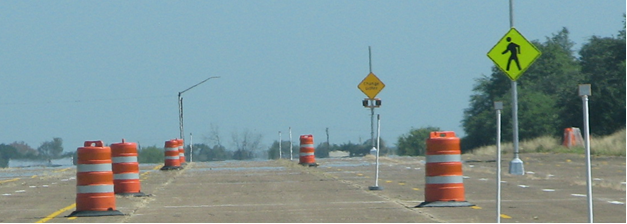
Figure 41. Photo. View of discomfort glare course.
The flash pattern used with the assemblies was selected based on FHWA official interpretation 4(09)-21 (I) released on June 13, 2012.(26) The flash pattern used with the circular and rectangular beacons in this closed-course study is shown in table 94. Figure 42 shows an oscilloscope reading of the light output for the rapid flash pattern. For the LED-embedded sign, the research team decided to use the same flash pattern used with the right or top beacon in the other assemblies. Table 95 shows the flash pattern used with the LED-embedded sign with
figure 43 showing the oscilloscope version.
Table 94. Rapid flash pattern.
Left or Bottom Beacon |
Right or Top Beacon |
Time Increment (milliseconds) |
On |
Off |
124 |
Off |
Off |
76 |
On |
Off |
124 |
Off |
Off |
76 |
Off |
On |
25 |
Off |
Off |
25 |
Off |
On |
25 |
Off |
Off |
25 |
Off |
On |
25 |
Off |
Off |
25 |
Off |
On |
25 |
Off |
Off |
25 |
Off |
On |
200 |
Total | 800 |
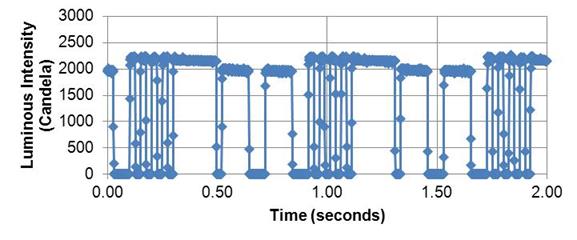
Figure 42. Graph. Flash pattern for rapid-flashing beacons.
Table 95. LED-embedded sign flash pattern.
LEDs |
Time Increment (milliseconds) |
Off |
400 |
On |
25 |
Off |
25 |
On |
25 |
Off |
25 |
On |
25 |
Off |
25 |
On |
25 |
Off |
25 |
On |
200 |
Total | 800 |
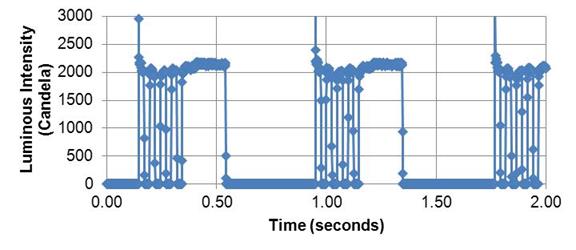
Figure 43. Graph. LED-embedded sign flash pattern (uses same five-pulse pattern as that used by the right beacon in a rapid flash pattern).
After the detection distance data and ramp-up data were collected, the light output for the beacons and LED-embedded signs were measured. To quantify the brightness of the pulsing lights, researchers used the TTI Photometric Range within the Visibility Laboratory. For each beacon and LED-embedded sign, a technician measured the 95th percentile peak intensity and the optical power of the device. For the discomfort glare devices, these measurements were taken at each of the six levels of the controller. The researcher took the measurements at a vertical angle of 0 degrees and a horizontal angle of 0 degrees. Figure 44 and figure 45 show examples of devices being measured.
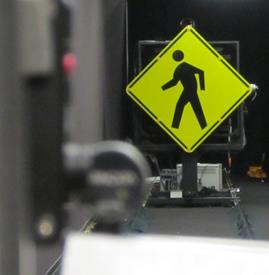
Figure 44. Photo. LED-embedded sign mounted on goniometer.
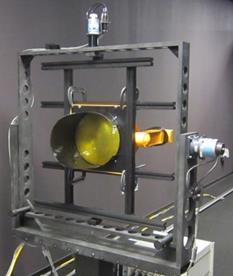
Figure 45. Photo. Mounted 12-inch circular beacon.
Figure 46 shows example of the peak intensity and the 95th percentile intensity. Peak luminous intensity is the maximum luminous intensity for a given flash. For the flash in figure 46, the peak intensity is 3,715 cd, which is 6.2 times greater than the Society of Automotive Engineers (SAE) standard J595 requirement for the center test point. The 95th percentile luminous intensity is the luminous intensity in which 95percent of the instantaneous intensity measurements are less than or equal to during the duration of the flash; instantaneous intensities measured during the dark period are not included in this measurement. The 95th percentile luminous intensity for the flash in figure 46 is 1,726cd, which is 2.9 times greater than the SAE standard J595 requirement for peak luminous intensity at the center test point.
Optical power is the integrated total of all flashes in a minute, in candela-seconds per minute (cd-s/min). Figure 46 shows an example where the flash has an integrated value of 836 cd-s. Within a minute, there are 58.7 of these flashes; therefore, the optical power is 49,073 cd-s/min, which is 3.4 times the SAE standard J595 minimum optical power for the center test point.
Figure 47 shows the measured optical power brightness for the devices used in the closed-course study. The measurements for those devices used in the driving portion have the same value (i.e., appear as a horizontal line) in the graph. The light intensity for these devices was not changed during the driving portion of the study. The two devices used in the discomfort glare portion of the study were changed from a setting of 1 to 6. The rectangular beacons had much higher optical powers compared with the other devices. Figure 48 shows the 95th percentile intensity brightness. Using 95th percentile intensity, the LED-embedded signs have similar values as the rectangular beacons.
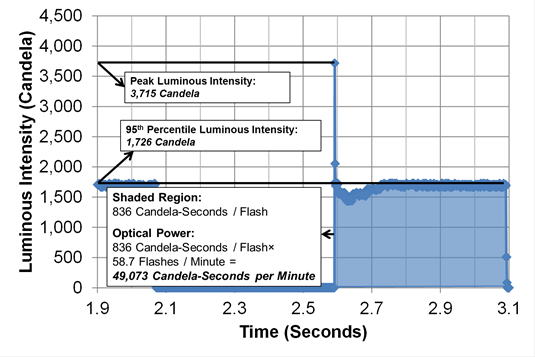
Figure 46. Graph. Example peak luminous intensity and optical power calculations.
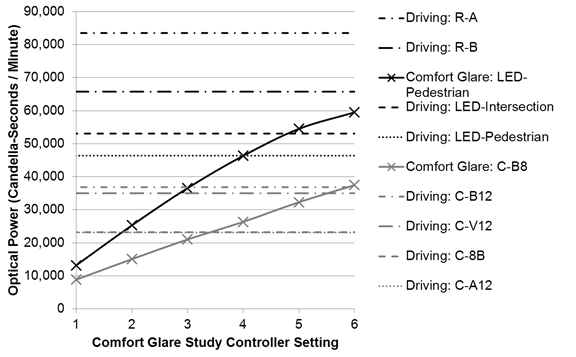
Figure 47. Graph. Optical power measurements for driving study assemblies compared with discomfort glare study controller settings (one setting in driving study).
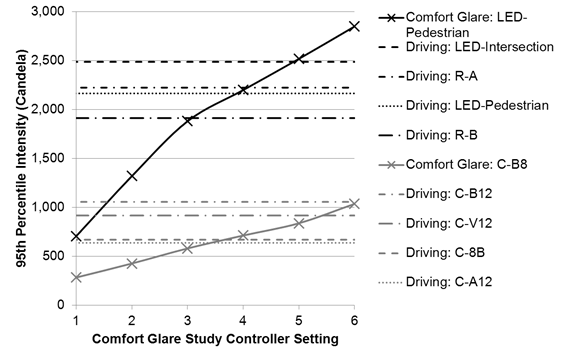
Figure 48. Graph. 95th percentile intensity measurements for driving study assemblies compared with discomfort glare study controller settings (one setting in driving study).
Stop Signs and Distractor Signs Selected for Driving Study
In addition to the study assemblies, distractor warning signs, distractor speed limit signs, and stop signs were included along the test route. The distractor signs served several purposes: to help hold participants’ attention on the course, to distract participants (to some extent) from the fact that the study assemblies were the focus of the research, and to provide some additional data points to observe detection and legibility distances. The stop signs were included on the course to ensure that the participants would stop at selected locations so that the researcher in the car could give instructions or make notes in the computer file.
The distractor signs included along the test route are listed in table 96, which also provides the average retroreflectivity reading for each sign. The retroreflectivity varies widely between the different stop and distractor signs; however, this was not a concern because these signs were not the focus of this study.
Three objects were used in the study: a small gray wooden box, a trash can, and a pedestrian in blue medical scrubs. The pedestrian was selected because the assemblies being tested are for pedestrian crossings. The small gray wooden box has been used in other TTI nighttime detection studies.(15) Desired was a third object that would be between a pedestrian and small box in height; a trash can was selected.
Objects could be located downstream of each study assembly and distractor sign. The research team decided that objects would not be located immediately downstream of the stop signs. Objects would also be located between signs so participants would need to look for objects at locations other than just when a sign was present. In most cases, if an object was present at a location in lap A, then an object was not present at that location in lap B. Table 97 lists the objects by position number for lap A and lap B. Figure 21 shows the positions for the objects.
The box was placed approximately 3 ft downstream of the sign (see figure 49). Because of the potential for the generator to block the view of the box when the box was used in conjunction with a study sign, the boxes were placed about 2 ft to the left of the sign post, which is approximately 11 ft from the edgeline of the lane. The dimension of the box was 9.25 inches at the widest point by 7.5 inches from the ground. Figure 50 provides a close-up of the box.
Table 96. Distractors and stop signs used in closed-course study.
Typea |
Sign faceb |
Lapc |
Positiond |
Retroe |
S |
STOP |
A&B |
S1 |
116 |
S |
STOP |
A&B |
S2 |
13 |
S |
STOP |
A&B |
S3 |
23 |
S |
STOP |
A&B |
S4 |
22 |
S |
Blank |
A&B |
S5 |
79 |
S |
STOP |
A&B |
S6 |
6 |
S |
STOP |
A&B |
S7 |
49 |
S |
STOP |
A&B |
S8 |
17 |
S |
STOP |
A&B |
S9 |
152 |
D |
Speed Limit 42 |
A |
D1 |
398 |
D |
Speed Limit 35 |
B |
D1 |
297 |
D |
Speed Limit B3 |
A |
D2 |
268 |
D |
Speed Limit 45 |
B |
D2 |
289 |
D |
Enough Silent |
A |
D3 |
488 |
D |
Energy Supper |
B |
D3 |
272 |
D |
Speed Limit 35 |
A |
D4 |
865 |
D |
Speed Limit F4 |
B |
D4 |
42 |
D |
Stop Ahead (Symbol) |
A |
D5 |
616 |
D |
Simple Design |
B |
D5 |
513 |
D |
Loose Gravel |
A |
D6 |
99 |
D |
Rough Road |
B |
D6 |
107 |
D |
Speed Limit 48 |
A |
D7 |
268 |
D |
Speed Limit Y2 |
B |
D7 |
256 |
D |
Speed Limit H8 |
A |
D8 |
226 |
D |
Speed Limit 25 |
B |
D8 |
28 |
D |
Train (Symbol) |
A |
D9 |
61 |
D |
Always Animal |
B |
D9 |
483 |
aType = either D for distractor signs or S for stop signs. The distractor signs had various sizes, backgrounds, and sheeting because these signs were obtained from previous studies. The stop signs were either 30- or 36-inch typical stop signs, except the sign at position S5. The sign at position S5 was alum high-intensity prismatic and did not have a legend (i.e., it was blank). The sheeting for the remaining stop signs varied because these signs were obtained from previous studies.
bSign Face = description of the face of the sign.
cLap = assembly included in either lap A or B of the driving portion on as part of the discomfort glare portion.
dPosition = location of the sign on the course (see figure 21).
eRetro = average of four retroreflectivity readings.
Table 97. Object location on course.
Object positiona |
Followsb | Lap A object | Lap B object |
O01 |
D1 | small gray box | empty |
O02 |
P1 | trash can | empty |
O03 |
D2 | empty | small gray box |
O04 |
P2 | empty | pedestrian |
O05 |
D3 | pedestrian | empty |
O06 |
D4 | empty | trash can |
O07 |
D5 | trash can | empty |
O08 |
P3 | small gray box | empty |
O09 |
D6 | empty | small gray box |
O10 |
P4 | trash can | pedestrian |
O11 |
After turn, S5 | small gray box | empty |
O12 |
After turn, S5 | empty | trash can |
O13 |
D7 | empty | empty |
O14 |
P5 | pedestrian | small gray box |
O15 |
After turn, S6 | small gray box | trash can |
O16 |
P6 | empty | small gray box |
O17 |
After turn, S7 | small gray box | empty |
O18 |
P7 | empty | small gray box |
O19 |
D8 | trash can | empty |
O20 |
D9 | empty | pedestrian |
O21 |
P8 | pedestrian | trash can |
aObject position = location of the object on the course (see figure 21).
bFollows = the position of the sign preceding the object or that the object is after a turn (see figure 21).
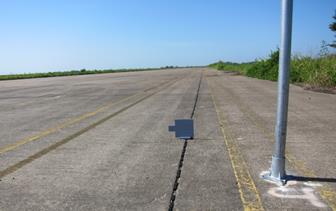
Figure 49. Photo. Small gray box on course.
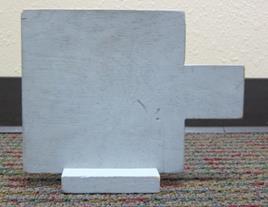
Figure 50. Photo. Close-up of small gray box.
The trash cans selected for this study were 42 inches high and 25 inches wide. The trash can was located 3 ft downstream of the sign (example shown in figure 51) when associated with a sign. Figure 52 shows an example of a pedestrian. Because of the length of the study and having three pedestrians present on each lap, several TTI staff members were used as pedestrians. The heights of the pedestrians ranged between 5 ft 2 inches and 6 ft 3 inches with the average height being 5ft 10 inches. The pedestrian stood approximately 3 ft downstream of the sign post and did not move as the participant approached the sign. Table 98 lists the study assemblies and distractor signs that preceded a pedestrian. Each of the study assemblies with circular or rectangular beacons was tested prior to a pedestrian at a minimum of one location. In some cases, the study assembly was tested prior to a pedestrian in two locations.
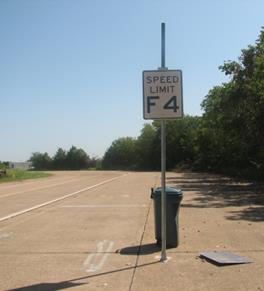
Figure 51. Photo. Trash can on course.
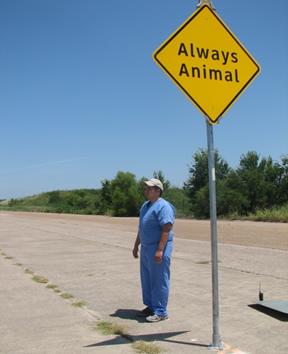
Figure 52. Photo. Pedestrian on course.
Table 98. Study device and sign face by object position (view distance in ft) used with pedestrians.
Study devicea | Sign faceb | Object | |||||
O04 (4308) |
O10 (4900) |
O14 (3965) |
O21 (3617) |
O05 (1452) |
O20 (2137) | ||
C-A12 | Trail Crossing | — |
— |
X |
X |
— |
— |
C-A12 | Wheelchair | — |
X |
— |
— |
— |
— |
C-B12 | Bike | X |
X |
— |
— |
— |
— |
C-B12 | Ped Crossing | — |
— |
X |
— |
— |
— |
C-B8 | Deer | — |
— |
— |
X |
— |
— |
C-B8 | Ped Crossing | X |
X |
— |
— |
— |
— |
C-V12 | Ped Crossing | X |
— |
— |
— |
— |
— |
C-V12 | Offset Intersection | — |
— |
— |
X |
— |
— |
R-A | Ped Crossing | — |
— |
X |
— |
— |
— |
R-A | Playground | — |
X |
— |
— |
— |
— |
R-B | Bikes & Ped | — |
— |
X |
X |
— |
— |
R-B | Ped Crossing | X |
— |
— |
— |
— |
— |
Distractor | Always Animal | — |
— |
— |
— |
— |
X |
Distractor | Enough Silent | — |
— |
— |
— |
X |
— |
aStudy Device = type of device, either distractor sign or study assembly where the beacons or LEDs included in the study assembly have C = circular, R = rectangular, A = above, B = beneath, V=both above and beneath, 12 = 12-inch circular beacon, 8 = 8‑inch circular beacon.
bSign Face = description of the symbol or text on the face of the sign used with the study device.
X = object (pedestrian) was present beyond the study device with the given sign face.
— indicates object (pedestrian) was not present beyond the study device with the given sign face.
Site Selection for Study Assemblies, Distractor Signs, Stop Signs, and Objects
Warning signs and speed limit signs were placed throughout the course as distractor signs. These additional signs helped to maintain participants’ attention and interest between assemblies, distracted participants from the study’s focus, and provided additional information regarding legibility distance (because drivers could not as easily guess at the words/numbers appearing on the distractor signs).
Figure 21 shows the route and the sign placements. Numbers on the graphic indicate the study assemblies (preceded by a “P”), distractor signs (preceded by a “D”), stop signs (preceded by an “S”), and objects (proceeded by an “O”), in the order in which they appeared on the route.
Table 99 lists the travel distance and viewing distance for each sign or object. Viewing distance is the distance between where a participant turned onto the tangent and where the sign or object was located. It represents the theoretical maximum detection distance. Because of how some drivers positioned themselves at a turn or stop sign, longer distances could be measured.
Position P7 on the course required the installation of the sign in the grass. The pre-existing lane markings are close to the edge of the concrete, which caused the sign to be located in the grass to maintain a 12-ft offset between the edgeline and the sign. The researchers selected the lightest study sign assembly for the in-ground installation at position P7.
The Riverside runways are used by many groups within Texas A&M University system and were experiencing high demand during the summer 2012. While groups were willing to share the facility, some accommodations had to be made in this study. The signs in positions P1, S1, and S6 had to be removed at the conclusion of the daytime session (about 4p.m.) and reinstalled prior to the start of the nighttime session (about 8 p.m.) to allow the landing of airplanes. Because a study assembly had to be removed every day, the researchers selected the assembly that would be easiest to remove, which was the sign with the embedded LEDs.
A previous study revealed that the position of a device on the runway could affect the detection distance.(25) Because beacons can be seen several thousand feet in advance of the device, the researchers decided to have one set of beacons on each straightaway on the course. This limited the study to a maximum of eight study assemblies with two of those assemblies in positions where the limited advanced viewing distances may affect the results. Therefore, the two study assemblies with lowest priority for the study—the without-beacon sign and the LED-embedded sign—were placed at these locations (positions P1 and P7) (see figure 21).
Another approach used to try to minimize the potential impact of position on the results was to rotate selected assemblies during the study. After data were recorded for a quarter of the participants, selected devices were rotated to a new position. With a goal of 64 total participants, researchers planned to rotate signs after data were collected for 16 (8 day and 8 night), 32, and 48 participants. The rotation typically took more than 5 hours to uninstall, transport, and reinstall the devices at their new location. Another benefit of rotation was the ability to gather detection to pedestrian data for different assemblies while the background to the pedestrian remained constant. While selected assemblies were rotated, the objects were not rotated. An object for a given lap was present for all participants. Because of the number of participants for the study (set based on available budget) and the desire to have a reasonable number of participants viewing the assemblies in each rotation, the researchers decided to have only four rotations. Given the number of assemblies included in the study, the preference would have been to have more rotations; however, all studies have limitations and this is recognized as one of the limitations for this study. The position for each of the assemblies is shown in table 99.
Several of the Riverside campus runways were already marked with yellow centerline striping and white edgeline striping to simulate rural roadways. Where striping was not present, the research team installed temporary raised pavement markers (RPM) to act as a roadway centerline.
Signs without beacons were installed using a base as shown in figure 53. Figure 54 shows the larger base used with study assemblies that had the increased weight of beacons or embedded LEDs. In one case (position 7), the sign was installed in the ground because the edgeline was too close to the pavement edge to install the sign on the concrete.
Table 99. Order of device presentation on closed course.
Order |
Positiona |
Travelb (ft) |
Viewc (ft) |
Groupd |
Order |
Positiona |
Travelb (ft) |
Viewc (ft) |
Groupd |
0 | Start | 0 | Start | Start |
24 |
O10 |
3 |
4,900 |
Object |
1 | D1 | 2,000 | 1,100 | Distract |
25 |
S5 |
380 |
5,280 |
Stop |
2 | O01 | 3 | 1,103 | Object |
26 |
O11 |
630 |
630 |
Object |
3 | P1 | 1,291 | 2,394 | Study |
27 |
O12 |
949 |
800 |
Object |
4 | O02 | 3 | 2,397 | Object |
28 |
D7 |
717 |
1,517 |
Distract |
5 | S1 | 363 | 2,759 | Stop |
29 |
O13 |
3 |
1,520 |
Object |
6 | D2 | 2,239 | 2,239 | Distract |
30 |
P5 |
2,442 |
3,962 |
Study |
7 | O03 | 3 | 2,242 | Object |
31 |
O14 |
3 |
3,965 |
Object |
8 | P2 | 2,064 | 4,306 | Study |
32 |
S6 |
371 |
4,336 |
Stop |
9 | O04 | 3 | 4,308 | Object |
33 |
O15 |
667 |
667 |
Object |
10 | S2 | 371 | 4,679 | Stop |
34 |
P6 |
1,521 |
2,188 |
Study |
11 | D3 | 1,449 | 1,449 | Distract |
35 |
O16 |
3 |
2,191 |
Object |
12 | O05 | 3 | 1,452 | Object |
36 |
S7 |
978 |
3,169 |
Stop |
13 | D4 | 1,379 | 1,379 | Distract |
37 |
O17 |
1,035 |
1,035 |
Object |
14 | O06 | 3 | 1,382 | Object |
38 |
P7 |
1,457 |
2,492 |
Study |
15 | D5 | 1,240 | 1,240 | Distract |
39 |
O18 |
3 |
2,495 |
Object |
16 | O07 | 3 | 1,243 | Object |
40 |
D8 |
1,229 |
1,229 |
Distract |
17 | P3 | 1,380 | 2,623 | Study |
41 |
O19 |
3 |
1,232 |
Object |
18 | O08 | 3 | 2,626 | Object |
42 |
S8 |
896 |
900 |
Stop |
19 | S3 | 230 | 230 | Stop |
43 |
D9 |
2,134 |
2,134 |
Distract |
20 | S4 | 2,449 | 2,449 | Stop |
44 |
O20 |
3 |
2,137 |
Object |
21 | D6 | 2,972 | 2,972 | Distract |
45 |
P8 |
1,477 |
3,614 |
Study |
22 | O09 | 3 | 2,975 | Object |
46 |
O21 |
3 |
3,617 |
Object |
23 | P4 | 1,922 | 4,897 | Study |
47 |
S9 |
1,620 |
5,237 |
Stop |
aPosition = location of the object (or sign) on the course (see figure 21).
bTravel = distance traveled from previous position (ft).
cView = distance (rounded) the item can be viewed after participant makes a turn (ft).
dGroup = start, stop, distract, study, or object. Start and stop represents the starting point or ending point of the course, distract represents the distractor signs. “Study” represents the study assemblies (see table 93), and “Object” represents the objects (see table 97).
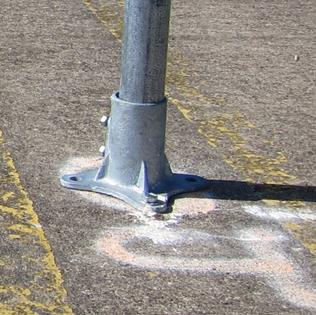
Figure 53. Photo. Base for signs without beacons/LEDs.
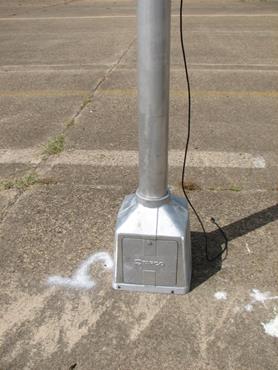
Figure 54. Photo. Base for signs with beacons/LEDs.
The study assemblies were to be located a minimum of 360 ft prior to the stop sign. The 360 ft represents the stopping sight distance for 45 mi/h. It was selected as a compromise to have enough distance before the end of the segment but also provide the largest amount of advanced viewing distance. The distance between the edgeline and the left edge of sign was 12 ft. The height between the pavement and the bottom of downward sloping arrow plaque was 6 ft minimum based on MUTCD Section 2A.18 (see paragraph 06, page 42, option for secondary signs to be 1 ft less than 7 ft).(2) The height between the pavement and the bottom of the diamondshaped sign was 7ft minimum.
The distractor and stop signs also had a distance of 12 ft between the edgeline and the left edge of the sign. The distance between the pavement and the bottom of stop and distractor signs was 6ft or 7ft minimum, respectively.
For all signs except the sign with embedded LEDs, the sign changes between laps were accomplished by lifting the sign up and out of the bottom bracket and then out of the top bracket (see figure 55 and figure 56). For the embedded LED sign, the bolts holding the sign onto the pole were removed, and the sign was exchanged. The stop signs were not changed between the laps, only the distractor signs and the study signs.
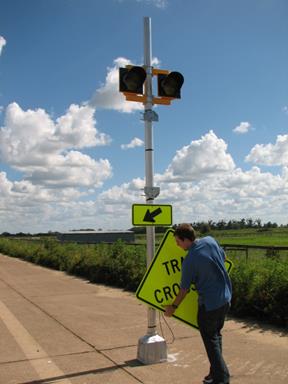
Figure 55. Photo. Example of sign change.
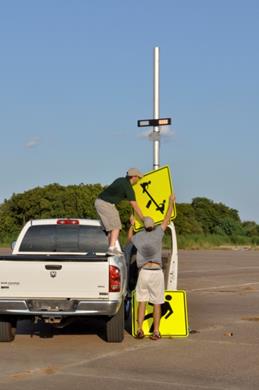
Figure 56. Photo. Another example of sign change.
A practitioner review of the assemblies and the course occurred prior to collecting the participant study data. Several engineers local to the area were invited to review the course during both daytime and nighttime conditions. In addition to another TTI staff member, an engineer from a nearby city reviewed the assemblies in a late afternoon and then returned after dark to review the course during nighttime conditions. The review confirmed that the study assemblies did represent how a city would have integrated the beacons with the sign. The review did not result in any changes to the study assemblies or the course. Another city engineer reviewed the study course in the initial days of data collection, again confirming that the devices used were appropriate.
The study was conducted under both daytime and nighttime conditions over 2 weeks in July/August 2012. The actual dates for the study were as follows:
- Wednesday, July 25, 2012 for practitioner review.
- Thursday, July 26–Friday, July 27, 2012.
- Monday, July 30–Friday, August 3, 2012.
- Sunday, August 5–Wednesday, August 8, 2012.
For July 26, 2012, sunset occurred at 8:24 p.m. On August 8, 2012, sunset occurred at 8:14 pm.
Initially, the arrival times for the participants were staggered with the idea that only one participant would be driving on the course at a time. That participant would drive the first lap, and members of the sign crew would follow behind the participant, changing signs in preparation for the second lap. This was the approach used successfully in previous studies. Because of the large number of sign and object changes required for this study, that approach resulted in large time gaps when the participant had to wait while sign changes and object changes were made and verified.
After the initial days of data collection, the TTI team developed a different approach for conducting the study. The revised approach required additional vehicles for members of the field crew along with extra objects to facilitate the placing and removing of objects; however, the benefits were a much faster study time for the participants and fewer staff needed to conduct the study (only 8 instead of 10 staff members). Another bonus for this approach was that couples could be scheduled for similar times. Previous experience has shown that older couples who participate in a study at night prefer having this option.
In the revised approach, used for the majority of the data collection, two participants drove the course a few minutes apart. The researcher in the test vehicle gave instructions to the participants that resulted in the participants being one stop sign (or straightaway) apart. For example, when the first participant was leaving S3, the second participant would be leaving S2. This ensures that the headlights of the following participant would not shine into the first participant’s rear view mirror and that the two participants would not cross paths at any point on the course. Both participants would wait between laps while the field crew changed signs and objects. When first implemented, it took the field crew slightly less than 20 min to change all the signs and objects. They quickly improved their time to less than 10 min for sign and object changes between laps.
The study took about 1.5 hours from meeting the participant to the participant receiving his or her payment (see table 100). Half of the participants drove during daylight hours and half during nighttime conditions. The following start times were used:
- 12:20 p.m.
- 2:20 p.m.
- 8:40 p.m.
- 10:10 p.m.
Table 100. Participant time in study.
Activity | Time (min) |
Escorting participant from front gate of Riverside to building where processing occurred | 5 |
Initial processing, training | 10 |
Drive to start, first lap | 20 |
Wait time for sign changes (time allocated for this task decreased as sign crew became more familiar with duties) | 10–20 |
Second lap, drive to discomfort glare location | 20 |
Discomfort glare task | 10 |
Final processing and payment | 5 |
Total | 80–90 |
The initial intent was to recruit a group of participants composed of one-quarter males over 55years, one-quarter females over 55 years, one-quarter males under 55 years, and one-quarter females under 55 years. Within each of those demographic groups, the goal was to have an even distribution between those who drove during the day and those who drove at night and between those who drove lap A first and those who drove lap B first. Therefore, the following divisions were used in structuring participant recruitment:
- Light level: day or night.
- Age group: young (younger than 55 years) and old (55 years or older).
- Gender: male or female.
- Lap driven first: A or B.
These divisions resulted in 16 participant categories. The research goal was to have 4 participants in each of the 16 categories, resulting in 64 participants. The study included 71 participants because participants were added to 1) replace participants whose data were not recorded successfully and 2) add additional data to offset missing data points not collected because signs were temporarily disabled or objects were not appropriately placed. The final participant pool is shown in table 101. Participants were at least 18 years old and possessed a valid driver’s license with no restrictions.
Table 101. Distribution of participants.
Age | Gender | Day | Night | Total | ||
Lap A first |
Lap B first |
Lap A first |
Lap B first | |||
Younger than 55 |
Female |
3 |
5 |
6 |
2 |
16 |
Male |
5 |
5 |
3 |
6 |
19 | |
55 or older |
Female |
4 |
5 |
5 |
4 |
18 |
Male |
6 |
2 |
3 |
7 |
18 | |
Total |
18 |
17 |
17 |
19 |
71 |
Participants were recruited by word of mouth, flyer distribution, and communication with people who participated in past studies and indicated an interest in future studies. Flyers with information about the study, location, contact information, dates, and compensation were distributed among friends and acquaintances and were posted in public places. Upon completion of the survey, participants received monetary compensation of $50.
The tasks for the participants while driving the closed course were to indicate when they could first perform the following:
- See warning lights.
- See road sign.
- Read the words or identify the symbol on the road sign.
- See object.
Two similar vehicles—2009 Ford Explorers—served as the participant cars for this experiment. The headlamps for these vehicles are 35 inches from the ground and 27 inches from center of the vehicle. Each vehicle was equipped with Qstarz BT-Q8181XT GPS receivers that reported the vehicle location at 10 Hz. The GPS receiver is a WAAS, EGNOS, and MSAS differential GPS device that uses WGS-84 datum with a position accuracy of 8.2 ft. Each receiver was affixed to the passenger side of the front windshield near the rear view mirror. TTI-developed software recorded the incoming GPS data stream into a text file along with single American Standard Code for Information Interchange (ASCII) character keyboard inputs from the researcher in the vehicle. The ASCII characters indicate responses by a participant during the study.
Participant intake was headquartered at TTI’s Environmental Emissions Research Facility on the Riverside campus. This location was selected because it was near the driving route, had public parking available, included restroom facilities, and was available for both daytime and nighttime use during the data collection period.
After meeting with a member of the research team to review the informed consent documentation and complete the demographic questionnaire, participants were given an overview of the study including how the data would be collected. They were also given a Snellen visual acuity test and the Dvorine color vision test.
To ensure consistency, the research team used scripts and slide shows to aid in providing instructions to each participant. The following script was used during intake:
- You will be driving a state-owned passenger vehicle on a closed course we have set up on airport runways, taxiway, and roadways here at the Riverside Campus. The vehicle is specially equipped to record and measure various driving characteristics, but drives just like a normal car. The researcher will be using a distance measuring instrument to mark various points as you drive. A researcher will be in the car with you at all times and will direct you when, where and how fast you will need to go. The fastest you will be asked to drive is 45 mi/h.
- Most of the route is marked with white and yellow striping just like you would see on an actual road. Part of the route is not striped, and when we reach these segments, the researcher will point you to the reflective pavement markings that will act as our road’s “center line.”
- While driving the test course you will see a number of road signs. There may be multiple signs on each stretch of road. As you approach each of the road signs, please tell the researcher:
- If you can see warning lights.
- When you can see the road sign.
- Then read the word or words on the sign as soon as you can read them. Some signs may not have words, and for these signs just tell me what you think the sign means. It is OK to make mistakes, just tell the researcher when you have made a mistake and the corrected word or words on the sign.
- Occasionally, you may see something at the edge of the road, such as a small box, a trash can, or a pedestrian. If you do see one of these, please tell me by saying “box,” “can,” or “pedestrian.”
- If you can see warning lights.
- We will drive the entire course twice. There will be a brief pause at the end of your first drive through the course to allow field personnel time to set up the study area.
- While we want you to focus on the road signs as accurately as possible, your most important job is to drive safely, always paying attention to the road ahead and keeping the test vehicle under control. In addition, while this is a restricted area and there should not be any other vehicles in our area, on occasion there are vehicles on the roadway. So you will need to pay close attention to other vehicles on the roadway and obey all STOP signs you see.
- Once in the study vehicle, a researcher will give you more specific instructions on the study procedures. If you do not have any questions, we will proceed to the study course. Do you have any questions?
A slide show on a laptop computer was used to illustrate the types of signs the participants might see. The slide show also included the three types of objects that would be placed on the course.
As part of the intake process, a computer measured the participant and experimenter’s response times to develop a correction factor for each driver. Along the course, the experimenter pressed a button when the participant indicated he or she saw lights, a road sign or an object, or read the words or identified the symbol on a road sign. There is a small lag between the participant speaking a word, e.g., “lights,” and the experimenter pressing the button. The lag could vary between the experimenters collecting the data. To address this concern, a pretest was developed to measure the lag time between when the participant saw a symbol on the computer screen and spoke the symbol’s name and when the experimenter pressed the button. The following four images were used in the exercise: down arrow, up arrow, plus sign, and black circle (or dot). Each symbol was repeated five times for a total of 20 random images. The task required the participant to identify which stimulus was present and say the correct word, a task analogous to the in-vehicle task of saying “lights” or “sign.” For the experimenters, the task was a simple reaction time test. They pressed a single button regardless of what the participant said, again analogous to the in-vehicle task.
The participant was instructed to say the name of the shape as quickly as possible once the image appeared on the computer screen. The experimenter pressed a button upon hearing the participant say the shape name. The software recorded the time difference between the shape appearing on the screen and when the button was pushed. The participant faced the computer screen, and the experimenter’s back was to the participant to avoid any anticipation on the part of the experimenter. Actual detection distance was determined from an average of the pretest reaction time for a participant along with the vehicle’s speed.
The participant was escorted to the instrumented vehicle and given a walk-through of the vehicle’s features. The participant was provided with the opportunity to adjust the seat and mirrors and to become accustomed to the controls of the vehicle.
Participants were informed that they would drive the instrumented vehicle on a closed course and were instructed to drive at a speed not exceeding 45 mi/h on the runways. They were asked to drive the runway system as though it were a regular roadway and were reminded that they had complete control of the vehicle at all times. A researcher accompanied the participant in the back seat, controlling the data collection equipment and providing direction. Participants were told to keep the vehicle’s headlamps on the low setting if testing at night. Conversation between the participant and the experimenter was kept to a minimum to ensure that the participant did not miss identifying any of the signs and lights on the course.
The participant drove the instrumented vehicle to the start position of the course, marked with an orange construction-zone barrel, waited while the researcher confirmed the lap (A or B) with the field crew and started recording a new data file on the computer, and then proceeded toward the first sign position.
The participant was to signify detection of the item by saying a preselected word to indicate the presence of warning lights, road sign, or object, and to read the words or identify the symbol on a road sign as soon as the sign was close enough to be legible. The experimenter recorded the response on the computer. The following instructions were given to the participants:
- Each time I tell you to start, you will do the following:
- Accelerate to a speed you are comfortable with, up to a maximum speed of 45mi/h.
- When you first see warning lights or a road sign on the right side of the road, I would like for you to say “lights” or “sign.” If you initially saw lights, as soon as you can see the road sign, tell me “sign.” Third, as soon as you are sure what the sign says, please read me the words or numbers on the sign. If you change your mind and see that the sign says something different than you initially thought, read me the new word or words. If a sign has no words on it, tell me what you think the sign means.
- If you see an object such as a box or trash can close to the edge of the roadway, I would like for you say “box” or “trash can.” If you see a pedestrian close to the edge of the roadway, please say “pedestrian.”
- The study course has several stopping points that we will use to provide you an opportunity to ask questions and rest, and provide me with an opportunity to give you further instruction if necessary.
- You may stop at any time if you are uncomfortable.
- Because you will be watching for a lot of signs, we won’t be able to carry on other conversation while you are driving.
- Please remember that safety comes first and follow all transportation and traffic laws.
- Accelerate to a speed you are comfortable with, up to a maximum speed of 45mi/h.
- If you don’t have any questions, we will start. Are you ready?
After completing the initial route, the participant was told to return to the orange construction barrel marking the route’s start point. Following notification from the sign crew that the signs had been changed from lap A to lap B (or vice versa), the researcher started a new data file and instructed the participant to proceed with the second lap. Following the second lap, the participant was directed to drive to the discomfort glare section of the course.
At the beginning of the discomfort glare study, researchers asked the participants to park 250 ft away from sign 1 (the first two orange barrels) (see figure 22 or figure 41). After the participant parked the vehicle, researchers turned on the beacon and asked the participant to indicate whether the brightness of the light was comfortable, irritating, or unbearable, which were defined as follows:
- Comfortable—when the glare is not annoying and the signal is easy to look at.
- Irritating—when the glare is uncomfortable; however, the participant is still able to look at it without the urge to look away.
- Unbearable—when the glare is so intense that the participant wants to avoid looking at it.
After the participant rated the first level of the controller, a technician increased the controller setting to level two. This process continued until the participant indicated the brightness was unbearable or the technician reached level six on the controller (the highest setting for the device). Figure 48 shows the brightness measurements for each device at the six controller levels; measurements were taken at vertical and horizontal angle of zero.
Once the participant indicated the brightness was unbearable or the technician reached level six, the researcher asked the participant to move to the next position. In order, the positions were the following:
- LED-embedded sign, 250 ft.
- LED-embedded sign, 150 ft.
- 8-inch circular beacons, 250 ft.
- 8-inch circular beacons, 150 ft.
This order remained the same for every participant, which is a limitation of this study.
Table 102 lists the demographic information for the 71 participants. The large number that selected retired for employment (29 percent) is a reflection of the emphasis on having half of the drivers over 55 years of age.
The response lag time between participants and researcher were determined for each participant during the intake procedure. The average response time for each participant in conjunction with the experimenter who was collecting data for that participant was determined. A review of the data revealed several potential errors. Very long response times were deemed to be caused by some distraction to the participant or the experimenter, which happened occasionally in the intake room. Very short response times were eliminated because, on occasion, the experimenter accidentally pressed the button before the participant spoke. To eliminate these outliers, data points that were outside of two standard deviations of the average response time for that participant were removed. These steps removed 83responses (about 6percent). Table 103 lists the average response time by experimenter before and after removing data. In general, the response time was about 1 s for any experimenter.
Table 102. Demographic information for 71 participants.
Characteristics |
Number (percent) | |
Gender | Male | 37 (52) |
Female | 34 (48) | |
Age Groups | < 55 | 35 (49) |
≥ 55 | 36 (51) | |
Race | Caucasian | 57 (80) |
African American | 1 (1) | |
Hispanic | 8 (11) | |
Not reported | 5 (7) | |
Employment | Full time | 26 (37) |
Part time | 13 (18) | |
Retired | 19 (27) | |
Not reported | 5 (7) | |
Student | 4 (6) | |
Other | 4 (5) | |
Miles Driven Per Year | < 10,000 | 19 (27) |
10,000–15,000 | 27 (38) | |
> 15,000 | 20 (28) | |
Not reported | 5 (7) | |
Normal Driving Conditions | Rural roads | 20 (28) |
Freeways | 3 (2) | |
City streets | 31 (44) | |
Mixed | 17 (26) |
Table 103. Response time by experimenter.
Researcher |
Number of responses |
Average response time (ms) |
Number of outliers (responses more than two standard deviations from average) |
Number of responses without outliers |
Average response time after outliers removed (ms) |
L | 499 |
972 |
28 |
471 |
931 |
C | 438 |
1,072 |
26 |
412 |
1,014 |
O | 320 |
947 |
20 |
300 |
906 |
J | 100 |
1,049 |
6 |
94 |
1,018 |
S | 40 |
1,071 |
3 |
37 |
1,038 |
All | 1,397 |
1,006 |
83 |
1,314 |
960 |
A more detailed review of the response time data indicates that adjusting the detection distance for response time should occur uniquely for each participant rather than using a per-experimenter average response time. Figure 57 shows the plot of the responses measured for each participant before eliminating the outliers. Figure 58 shows the plot of the responses measured after eliminating the outliers. As can be seen in the plots, some participants had average response time below 0.8 s, while other participants’ response times averaged above 1.2 s. Therefore, the average response time by participant rather than by experimenter was used to adjust the detection distance.
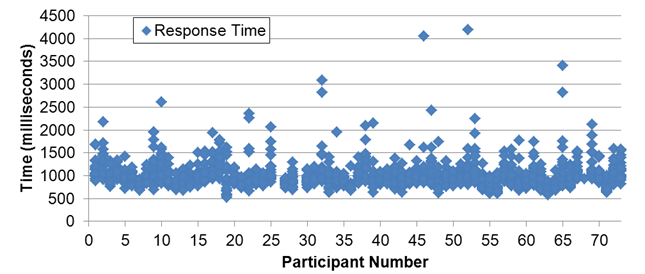
Figure 57. Graph. Measured response times by participant.
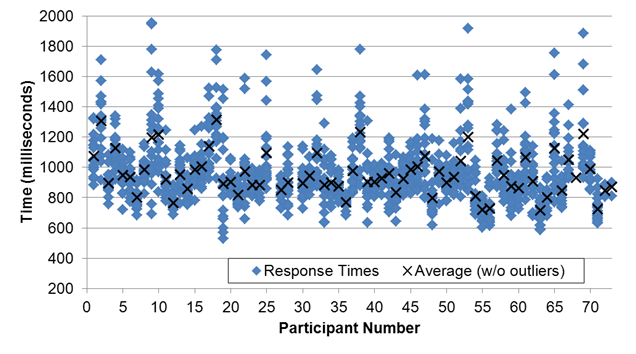
Figure 58. Graph. Response times by participant after removing outliers.
The measured detection distance was adjusted using the average response time for the participant and the speed of the vehicle at the point when the participant responded to a light or sign. The adjusted detection distance was an average of 2 percent higher than the GPS-based detection distance across all participants and sign/object locations. When the detection distances was shorter, such as to objects or reading the sign face, the average adjustment was about
4 to 6percent. The maximum response time adjusted distance was 47 ft, while the minimum was 0 ft, a situation that occurred when the vehicle was stationary.
The computer software program along with the GPS unit were used to determine the velocity and GPS coordinates when the driver identified a light, sign, or object, or when the participant read the sign. The data are recorded in a spreadsheet that contains a continuous stream of time, GPS coordinates, and velocity data. The time, velocity, GPS coordinates when the driver said “light,” “sign,” or “object,” or read the words on the sign were marked in the data streams.
The GPS locations of each of the signs and objects were recorded before the study began. The detection distance was determined by subtracting the location of the sign or object from the locations marked by the experimenter in the vehicle. This calculated distance was then adjusted to account for the response time of the experimenter and participant. Average response time of the experimenter and specific participant was multiplied by the vehicle’s velocity at the time of identification to obtain the response distance. The response distance was added to the detection distance to obtain the adjusted detection distance. The adjusted detection distance was used in all the analyses.
For some analyses, results are presented visually, in the form of box-plots, or quantitatively, in the form of statistical analysis. Box plots presented in this report were generated using the convention that the central line in the “box” represents the median data point (see figure 59). The top of the box represents the 75th percentile and the bottom represents the 25th percentile. Thus, the relative position of the median score within the 75th and 25th percentiles can give some indication about the skewness of the data. The “whiskers” represent the data that lay 1.5times beyond the interquartile range (IQR). This is the range between the 25th and 75th percentiles. If all data below the 25th percentile and above the 75th percentile are within 1.5times the IQR, then the end of the whisker represents the greatest or smallest value. Otherwise, all outliers beyond 1.5 times the IQR, added or subtracted from the 25th and 75th percentiles, respectively, are plotted using small black circles.
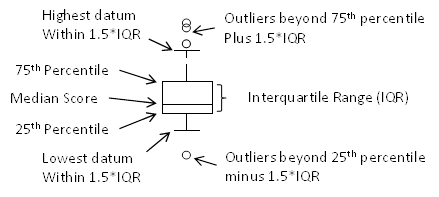
Figure 59. Diagram. Box plot details.
Before proceeding with the statistical analyses, the data were reviewed in a search for records with duplicate information regarding the participant, and miscoded information regarding the response type, lap number, specific position of assembly or sign face presented in the assembly. These records typically generated duplicate data points that were corrected later. For example, a participant said “Word,” but after a few seconds said “Word” again for the same sign. After identifying all 262 instances (about 2 percent of the data stream), they were removed from further analysis.
As a second stage of data cleaning, extreme data points were identified from various box-plot representations of the data as potential outliers whenever their distance from the mean exceeded 2.5 standard deviations (roughly corresponding to the 99.38th percentile of a normal distribution). Some of these early candidates were identified as anomalous and removed at that point. For example, for participants who were perceived as guessing at a long distance from the signs, the first stage of the data cleaning could not remove the instances when they guessed correctly, but in the second stage they appeared as extremely long distances.
A final stage of this process consisted of observing outlying data points after performing the statistical analysis. In addition, some data points were identified in the second stage of data cleaning and therefore were removed at this stage. Eighteen data points were removed from the second and third stages of data cleaning.
As expected, the detection distance to the beacons/LEDs was large. During the daytime, the average detection distance to the assemblies with beacons or LEDs was 2,244 ft. At night, the average detection distance was even longer, 3,236 ft. In several cases, the “light” detection distance was limited by the amount of viewing distance to the assembly. Therefore, the detection distances reported here could be shorter than the distance that a driver could detect a blinking beacon or LED. The conditions of the closed-course study; however, should result in very long detection distances. The participants were told to look for lights, and there were minimal background elements, such as billboards or street activity, competing for a driver’s attention.
The average detection distance to the sign (i.e., when the participants stated that they could see a sign) by type of sign (study assembly, distractor, or stop) along with age group and night or daytime conditions is shown in table 104. Caution is offered with the data because there are several elements that affect the results, such as viewing distance, position on the course, and the preceding sign or assembly. The focus of this study was legibility (i.e., the distance to read the study sign) and detection of the object located beyond a study sign. Therefore, the researchers did not attempt to control for those elements that could affect the detection of a sign and only presented averages and general observations regarding sign detection. The presence of beacons/LEDs along with the better quality retroreflectivity appears to contribute to the ability of the participants to see the sign from a greater distance when comparing the results for the assemblies (nighttime detection distance of 1,855 ft) with the results for the distractor signs (nighttime detection distance of 1,301 ft). Detection to the stop signs was lower than detection to other signs; however, there was always another sign located close to the stop sign, so the shorter detection distance may be affected by the presence of the other signs. Note that the average detection distances for all the sign types are greater than the stopping sight distance for 45 mi/h (360 ft). The results in table 104 do reveal a difference owing to old versus young; however, a difference by daytime versus nighttime is not as evident. Overall, the participants had a slightly longer sign detection distance during the day compared with the night.
Table 104. Sign detection distance by sign type without attempts to control for external elements such as viewing distance that could affect results.
Old or Young |
Sign type |
Daytime |
Nighttime |
||||
Count |
DD (ft) |
SD (ft) |
Count |
DD (ft) |
SD (ft) |
||
Old | Assembly |
262 |
1,925 |
782 |
219 |
1,814 |
921 |
Old | Distractor |
314 |
1,121 |
420 |
297 |
1,235 |
523 |
Old | Stop |
288 |
737 |
460 |
280 |
834 |
540 |
Old Total |
864 |
1,237 |
743 |
796 |
1,253 |
765 |
|
Young | Assembly |
262 |
2,311 |
829 |
284 |
1,886 |
733 |
Young | Distractor |
303 |
1,277 |
447 |
338 |
1,360 |
551 |
Young | Stop |
291 |
1,135 |
796 |
336 |
1,083 |
789 |
Young Total |
856 |
1,545 |
871 |
958 |
1,419 |
768 |
|
Assembly |
524 |
2,118 |
828 |
503 |
1,855 |
820 |
|
Distractor |
617 |
1,198 |
440 |
635 |
1,301 |
541 |
|
Stop |
579 |
937 |
680 |
616 |
970 |
697 |
|
Grand Total |
1,720 |
1,390 |
823 |
1,754 |
1,344 |
771 |
Count = number of participants.
DD = average detection distance (ft).
SD = standard deviation detection distance (ft).
One of the objectives of this study was to determine whether sign legibility distance to a study assembly is affected by the shape, size, or placement of the beacon/LEDs. The preliminary evaluations revealed confounding issues between the sign face and the beacon type, which is not surprising because each beacon type had only two sign faces. To handle this issue in the analysis, the following two approaches were used:
- Pedestrian Crossing Sign Only Data: This approach focused on the findings when the Pedestrian Crossing sign was used in an assembly.
- All Assemblies Data: This approach included a new variable to group the sign faces into one of three groups so that the data for all assemblies could be considered. The following groups were identified for the new variable “sign.fam” (for sign family):
- Ped X-ing sign: Pedestrian Crossing sign (W11-2).
- Other common signs: Deer (W11-3), T-Intersection Ahead (W2-2), and Offset Intersections (W2-7L).
- Uncommon signs: Bike (W11-1), Playground (W15-1), Wheelchair (W11-9), Trail Crossing (W11-15a), and Ped and Bike (W11-15).
To show the range of legibility distance being considered, table 105 lists the average and standard deviation of the legibility distance for daytime and nighttime. The analyses were separated into daytime and nighttime because 1) daytime and nighttime detection distances differed considerably and 2) to permit the opportunity to determine whether variables were affecting legibility distances differently in day and night conditions.
Table 105. Legibility distance by assembly type and sign face.
Assembly | Sign face | Daytime | Nighttime | ||||
Count |
DD (ft) |
SD (ft) |
Count |
DD (ft) |
SD (ft) | ||
A: C-A12 | Trail Crossing | 34 | 284 | 100 | 34 | 226 | 89 |
A: C-A12 | Wheelchair | 35 | 714 | 350 | 35 | 538 | 206 |
A: C-B12 | Bike | 34 | 617 | 199 | 36 | 602 | 467 |
A: C-B12 | Ped X-ing | 35 | 993 | 410 | 36 | 786 | 236 |
A: C-B8 | Deer | 34 | 1,007 | 397 | 36 | 643 | 279 |
A: C-B8 | Ped X-ing | 35 | 1,002 | 330 | 35 | 724 | 263 |
A: C-V12 | Offset Intersection | 34 | 973 | 381 | 35 | 777 | 282 |
A: C-V12 | Ped X-ing | 36 | 1,028 | 320 | 36 | 780 | 284 |
A: LEDs | Offset Intersection | 35 | 970 | 423 | 35 | 822 | 352 |
A: LEDs | Ped X-ing | 34 | 1,020 | 290 | 35 | 839 | 332 |
A: R-A | Ped X-ing | 33 | 994 | 360 | 35 | 708 | 190 |
A: R-A | Playground | 35 | 536 | 294 | 36 | 377 | 204 |
A: R-B | Bike & Ped | 34 | 535 | 276 | 33 | 423 | 259 |
A: R-B | Ped X-ing | 34 | 980 | 377 | 36 | 672 | 221 |
A: WO-B | Ped X-ing | 34 | 1,061 | 437 | 35 | 784 | 233 |
A: WO-B | T-Intersection | 35 | 1,102 | 414 | 36 | 886 | 326 |
Grand Total | 551 | 864 | 415 | 564 | 663 | 326 |
Count = number of participants.
DD = average detection distance (ft).
SD = standard deviation detection distance (ft).
Each dataset was analyzed using linear mixed effects models (LMM). These kinds of models combine characteristics from both linear regression and analysis of variance (ANOVA) with replication. The model was specified such that the data structure, known associations between variables, and systematic variation in the response variable were appropriately accounted. The analysis treated the co-dependency of data points from different drivers by explicitly adding a random effect for each participant in the experiment. The order of the laps was also treated this way. Within the blocking structure described above (i.e., light condition/driver/lap number), the analysis incorporated fixed effects for other variables of interest. In the case of legibility distance, the fixed effect variables were age group, sign family, and type of assembly. Estimates, confidence intervals, and conclusions were later extracted for these effects.
Finally, the analysis applied weights in inverse proportion to the estimated travel time between the point of detection and the assembly. This adjustment was necessary because data showed larger variability at greater distances from the assemblies. (This condition is known as heteroskedasticity in the statistics literature.) Preliminary examinations considered several other variables, such as position on the course and view distance. For some of the analyses, interactive terms were included to account for interaction between variables. All statistical analyses were performed using open source data analysis packages. (See references 27 through 30.)
It was necessary to adjust the model standard errors when comparing various pairs of assemblies. In such instances, the confidence in the model estimates decreases as the number of comparisons increases. This occurs because the chance of a type-I error is greater for simultaneous comparisons than for single comparisons.
A similar adjustment was made when comparing groups of assemblies. The reason given above is valid in this case too, but more important, the adjustment also permits accounting for correlation among the model estimates, which greatly affects the standard error of group comparisons. An example of group comparisons is investigating for a difference between all assemblies with rectangular and all assemblies with circular beacons.
Daytime Legibility Distance
An objective of this study was to investigate whether the shape of the beacons (circular or rectangular), the size of the beacon (e.g., 12 inches or 8 inches), or the placement of the beacon (e.g., above or below the sign) affected the results.
Table 106 shows the LMM results, and table 107 shows the ANOVA results for daytime. Preliminarily, the estimates for the effects of particular assemblies do not seem statistically significant (compared with their standard errors). This observation is consistent with the ANOVA results for the model (showed at the end of the table) where Assembly as a group is a statistically insignificant factor to explain legibility distance variation (p-value of 0.0565).
Table 108 shows the daytime pair comparison results from the model in table 106. The shape of the beacon—circular or rectangular—did not have an effect on legibility distance as shown by the non-significant result for the paired comparison of circular to rectangular. The individual pair comparisons of C-B12 to R-B and R-A to C-A12 were also not statistically significant, indicating that the daytime legibility distances are similar for each pair. The comparison of the legibility distance with comparison of size of beacon (i.e., C-B8 and C-B12) also did not show a significant difference between legibility distances.
The evaluation that examined legibility when the beacons were placed above or below the signs found no significant difference in distances. When reviewing the comparisons of individual pairs, the legibility distance for R-A and R-B were similar; however, C-B12 and C-A12 were different (statistically significant) (see table 108). This finding is additional evidence that the uncommon signs are confounding the results. When the circular beacons were located below the sign, drivers could read the signs at a greater distance upstream than when beacons were located above the sign. The assemblies with the 12-inch circular beacons above the sign had uncommon signs (Trail Crossing and Wheelchair) while the assemblies with the circular beacons below the sign had the more familiar signs of Ped X-ing and Bike, which confounds the validity of this finding. To offset this limitation, only the data when the Pedestrian Crossing sign was present was examined.
Table 106. Linear mixed-effects model results using all assemblies for daytime legibility distance.a
Variable | Value | Std. error | DF | t-value | p-value |
Reference Levelb | 560.6991 | 55.77835 | 490 | 10.05227 | 0.0000 |
O.Y: young | 531.4206 | 63.2773 | 33 | 8.398282 | 0.0000 |
Sign.Fam: Ped X-ing | 92.5412 | 36.11634 | 490 | 2.562309 | 0.0107 |
Sign.Fam: Uncommon | -216.2926 | 46.45764 | 490 | -4.655694 | 0.0000 |
Type_AsA: C-A12 | -54.663 | 30.64454 | 490 | -1.783778 | 0.0751 |
Type_AsA: C-B12 | 68.5406 | 35.13588 | 490 | 1.950728 | 0.0517 |
Type_AsA: C-B8 | 73.4926 | 46.41126 | 490 | 1.583508 | 0.1140 |
Type_AsA: C-V12 | 72.4622 | 46.20551 | 490 | 1.568259 | 0.1175 |
Type_AsA: LEDs | 56.3263 | 47.01232 | 490 | 1.198118 | 0.2315 |
Type_AsA: R-A | 6.2942 | 33.8891 | 490 | 0.185729 | 0.8527 |
Type_AsA: WO-B | 89.4155 | 47.13876 | 490 | 1.896858 | 0.0584 |
O.Y: young X Sign.Fam: Ped X-ing | -37.1006 | 57.0205 | 490 | -0.650654 | 0.5156 |
O.Y: young X Sign.Fam: Uncommon | -279.2907 | 52.69738 | 490 | -5.299899 | 0.0000 |
aNotes:
- Linear mixed-effects model fit by REML.
- Fixed effects: Adj_Dis ~ O.Y + Sign.Fam + Type_As + O.Y X Sign.Fam.
- O.Y. (age: old or young) = older driver.
- Sign.Fam (sign family) = common signs.
- Type_AsA (assembly type, see figure 23 to figure 38) = R-B.
DF = Degrees of Freedom.
Table 107. ANOVA results using all assemblies for daytime legibility distance.
Variable | numDF | denDF | F-value | p-value |
(Intercept) | 1 | 490 | 789.9444 | < .0001 |
O.Y | 1 | 33 | 60.4778 | < .0001 |
Sign.Fam | 2 | 490 | 284.1375 | < .0001 |
Type_As | 7 | 490 | 1.9768 | 0.0565 |
O.Y X Sign.Fam | 2 | 490 | 21.9561 | < .0001 |
Table 108. Daytime legibility distance multiple comparison using all assemblies data.
Test |
Item |
Comparison |
Estimate |
Std. error |
Z-value |
Pr(>|z|) a |
Simultaneous Tests for General Linear Hypotheses |
Place |
(Above) - ( Below) = 0 |
-58.455 |
24.211 |
-2.414 |
0.08335 . |
Shape |
(Circular) - (Rectangular) = 0 |
3.792 |
23.555 |
0.161 |
0.99978 | |
Size |
(C-B8 ) - (C-B12) = 0 |
4.952 |
46.579 |
0.106 |
0.99996 | |
Confirmation (Individual Pair Comparisons) | Shape |
(C-B12 ) - (R-B) = 0 |
68.541 |
35.136 |
1.951 |
0.23267 |
(R-A) - (C-A12) = 0 |
60.957 |
33.396 |
1.825 |
0.29364 | ||
Place |
(R-A) - (R-B) = 0 |
6.294 |
33.889 |
0.186 |
0.9996 | |
(C-B12 ) - (C-A12) = 0 |
123.204 |
35.553 |
3.465 |
0.00334** |
aSignificance values are as follows: p < 0.10; * p < 0.05; ** p < 0.01; and *** p < 0.001.
When the evaluation only considered those conditions when the pedestrian crossing sign was present, no significant differences between assemblies (LEDs, WO-B, R-B, R-A, C-V12, C-B8, C-B12) were identified. Stated in another manner, the presence of a yellow rapid-flashing beacon did not affect the legibility distance to the pedestrian crossing sign during daytime conditions.
Nighttime Legibility Distance
The linear mixed-effects model for the nighttime legibility distance when using the data for all assemblies (see table 109) shows that the following variables are significant: age (old, young), sign family, assembly type, and interaction between age and sign family. Assembly type was not significant at the 0.05 level in the daytime model while it was in the nighttime model. This suggests assembly type influences sign legibility at night more than it does during daytime conditions. Table 110 shows the ANOVA results.
Table 111 shows the results for the nighttime comparisons regarding the shape, size, and placement of beacon. As shown in table 111, the circular beacons were associated with longer legibility distances as compared to rectangular beacons. Examining the pairs of circular to rectangular beacons in similar positions revealed that the shape of the beacons was not significant when the beacons were above the sign (see R-A compared with C-A12); however, the shape of the beacons was significant when the beacons were below the sign (see C-B12 with R‑B). The participants read the sign 157 ft earlier when the beacon was a 12-inch circular beacon as compared to a rectangular beacon located below the sign.
The comparison of the legibility distance for size of beacon (i.e., C-B8 and C-B12) also shows a significant difference between legibility distances. Drivers were able to read the sign earlier with 12-inch lenses compared with 8-inch lenses.
The placement of the beacon above or below the sign was also significant; however, examining the specific pair comparisons reveals no significant difference between R-A and R-B and a significant difference between CA-12 and CB-12. Therefore, this comparison may be compromised by the sign choice for C-A12.
Table 109. Linear mixed-effects model results using all assemblies for nighttime legibility distance.a
Variable | Value | Std. error | DF | t-value | p-value |
Reference Levelb | 479.2589 | 51.06080 | 501 | 9.386044 | 0.0000 |
O.Y: young | 294.0365 | 59.69037 | 34 | 4.926029 | 0.0000 |
Sign.Fam: Ped X-ing | 60.1758 | 29.94397 | 501 | 2.009615 | 0.0450 |
Sign.Fam: Uncommon | -243.4851 | 36.84204 | 501 | -6.608892 | 0.0000 |
Type_AsA: C-A12 | 4.2572 | 24.39833 | 501 | 0.174495 | 0.8615 |
Type_AsA: C-B12 | 156.5475 | 27.49044 | 501 | 5.694617 | 0.0000 |
Type_AsA: C-B8 | -18.2307 | 34.48298 | 501 | -0.528688 | 0.5973 |
Type_AsA: C-V12 | 59.7811 | 35.08480 | 501 | 1.703902 | 0.0890 |
Type_AsA: LEDs | 95.5236 | 36.33491 | 501 | 2.628975 | 0.0088 |
Type_AsA: R-A | 16.0609 | 24.88700 | 501 | 0.645354 | 0.5190 |
Type_AsA: WO-B | 145.0945 | 36.24990 | 501 | 4.002619 | 0.0001 |
O.Y: young X Sign.Fam: Ped X-ing | -99.4175 | 41.93428 | 501 | -2.370793 | 0.0181 |
O.Y: young X Sign.Fam: Uncommon | -144.0525 | 38.82845 | 501 | -3.709974 | 0.0002 |
aNotes:
- Linear mixed-effects model fit by REML.
- Fixed effects: Adj_Dis ~ O.Y + Sign.Fam + Type_As + O.Y:Sign.Fam
- O.Y. (age: old or young) = older driver.
- Sign.Fam (sign family) = common signs.
- Type_AsA (assembly type, see figure 23 to figure 38) = R-B.
Table 110. ANOVA results using all assemblies for nighttime legibility distance.
Variable |
numDF |
denDF |
F-value |
p-value |
(Intercept) | 1 | 501 | 405.479 | < 0.0001 |
O.Y | 1 | 34 | 15.1728 | 0.0004 |
Sign.Fam | 2 | 501 | 313.0319 | < 0.0001 |
Type_As | 7 | 501 | 9.6389 | < 0.0001 |
O.Y X Sign.Fam | 2 | 501 | 6.9101 | 0.0011 |
Table 111. Nighttime legibility distance multiple comparisons using simultaneous tests for general linear hypostheses.
Groupa |
Item |
Linear Hypotheses |
Estimate |
Std. error |
z-value |
Pr(>|z|) b |
ALL | Place |
(Above) - ( Below) = 0 |
-68.11 |
18.65 |
-3.651 |
0.0017** |
ALL | Shape |
(Circular) - (Rectangular) = 0 |
72.37 |
18.08 |
4.002 |
< 0.001*** |
ALL | Size |
(C-B8) - (C-B12) = 0 |
-174.78 |
35.70 |
-4.896 |
< 0.001*** |
IPC | Shape |
(C-B12) - (R-B ) = 0 |
156.55 |
27.49 |
5.695 |
< 0.001*** |
IPC | Shape |
(R-A) - (C-A12) = 0 |
11.80 |
24.33 |
0.485 |
0.9835 |
IPC | Place |
(R-A) - (R-B) = 0 |
16.06 |
24.89 |
0.645 |
0.9538 |
IPC | Place |
(C-B12) - (C-A12) = 0 |
152.29 |
27.76 |
5.487 |
< 0.001*** |
Sign | Assembly |
(R-A) - (WO-B ) = 0 |
-74.58 |
37.97 |
-1.964 |
0.12317 |
Sign | Assembly |
(R-B) - (WO-B ) = 0 |
-123.70 |
37.64 |
-3.287 |
0.00293** |
Sign | Assembly |
(C-B8) - (WO-B ) = 0 |
-84.96 |
37.92 |
-2.240 |
0.06509 |
aGroup:
- ALL = all assemblies data.
- IPC = All Assemblies Data: Confirmation (Individual Pair Comparisons).
- Sign = Pedestrian Crossing Only Data (i.e., Data for Assemblies when Pedestrian Crossing Sign is Present).
Box plots were generated to aid in the review of the Pedestrian Crossing sign data. Figure 60 shows the legibility distance for young drivers to assemblies with a pedestrian crossing symbol, while figure 61 is a similar plot for old drivers. The average legibility distance was similar between the R-B and the C-B8 or the R-A for both young and old drivers. In the remaining cases, the average legibility distance for R-B was less than the legibility distance for C-B12, C-V12, LEDs, and WO-B.
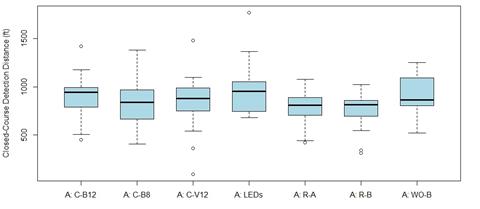
Figure 60. Graph. Box plots for nighttime legibility distance for assemblies with pedestrian crossing sign for young participants.
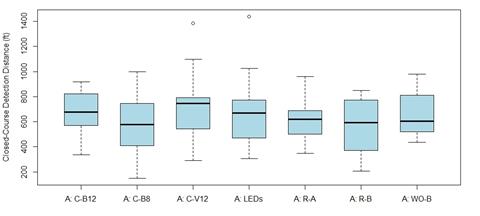
Figure 61. Graph. Box plots for nighttime legibility distance for assemblies with pedestrian crossing sign for old participants.
The evaluation that considered only those conditions when the Pedestrian Crossing sign was present again confirms the finding that the yellow rapid-flashing beacon is affecting drivers’ ability to read signs. Table 112 contains the results of the statistical model and shows that the following variables were significant: age (young or old), R-A, R-B, and C-B8. Next, the pair comparisons were done for these beacons (see table 111). The pair comparison showed that the difference between R-A and WO-B was not significant, that the difference between C-B8 and WO-B was significant at a p-value less than 0.1, and that the difference between R-B and WO-B was significant at a p-value less than 0.05. Stated in another manner, the presence of the RRFB located below the sign affected the legibility distance to the Pedestrian Crossing sign during nighttime conditions. Drivers were 124 ft closer to the sign before they could read the symbol compared with the distance when they could read the symbol when an RRFB was not present.
Table 112. Linear mixed-effects model results for assemblies with pedestrian crossing sign for nighttime legibility distance.a
Variable |
Value |
Std. error |
DF |
t-value |
p-value |
Reference Levelb |
664.5759 |
49.31322 |
206 |
13.476627 |
0.0000 |
O.Y: young |
208.1935 |
58.40199 |
34 |
3.564836 |
0.0011 |
Type_AsA: R-A |
-72.9047 |
38.09806 |
206 |
-1.913607 |
0.0571 |
Type_AsA: R-B |
-112.0146 |
37.26261 |
206 |
-3.006086 |
0.0030 |
Type_AsA: C-B12 |
2.7815 |
38.06915 |
206 |
0.073063 |
0.9418 |
Type_AsA: C-B8 |
-80.8886 |
37.88780 |
206 |
-2.13495 |
0.0339 |
Type_AsA: C-V12 |
-29.944 |
37.89882 |
206 |
-0.790103 |
0.4304 |
Type_AsA: LEDs |
20.2158 |
38.81704 |
206 |
0.520798 |
0.6031 |
aNotes:
- Linear mixed-effects model fit by REML.
- Fixed effects: Adj_Dis ~ O.Y + Type_As.
- O.Y. (age: old or young) = older driver.
- Type_AsA (assembly type, see figure 23 to figure 38) = WO-B.
Key Findings Regarding Legibility Distance
For the analysis that focused on the legibility distance, which is the distance between the sign and the participant when the participant reads the message on the sign, results indicate the following:
- As expected, the legibility distance for signs during the day is greater than the legibility distance for signs at night.
- Younger driver legibility distance is greater than older driver legibility distance. Previous studies on crosswalk markings and on stop signs with LEDs found age to be not significant.(25,31) In those studies, the participant did not have to “read” a sign. In the crosswalk marking study, the participant indicated when they could see the markings. For stop signs, the stop sign’s shape and color are more important than reading the word “stop.” Finding age to be significant indicates that future studies of beacons may need to focus on older participants explicitly.
- The symbol or word(s) on the sign face confounds the results. When the study was planned, different signs were desired to provide variety during the study. The researchers were concerned that the driver would always anticipate a pedestrian crossing sign when he or she saw a beacon, so alternative signs were needed. The researchers selected signs that could be associated with a pedestrian crossing, such as pedestrian crossing, trail crossing, bike, playground, bike & ped, and wheelchair. The researchers also selected signs that had a look similar to the pedestrian crossing sign such as the deer crossing, t‑intersection, and offset intersection signs. Because the type of sign confounds the results, understanding how the beacons/LEDs influence the detection distance is more complex. A new variable, sign family, was added to the evaluation to reflect whether the symbol is common or uncommon. In the sign family variable, the signs were grouped into the following three classes: pedestrian crossing, other common signs (deer, t-intersection, offset), and uncommon signs (wheelchair, trail crossing, bike, playground, and bike & ped). Finding that the sign face is significant in this study indicates that the profession needs to be concerned with the messages placed on a sign when the sign is used with beacons/LEDs. The estimated closed-course legibility distance to an uncommon sign (e.g., trail crossing) for an older driver at night was 236 ft, which while greater than the MUTCD estimation of legibility distance for a sign with 6-inch letters, is much less than the legibility distance for the symbol signs.
- During the nighttime, drivers were able to read the sign earlier with 12-inch lenses compared with 8-inch lenses. During daytime, the distance to read the sign was similar for 12- and 8-inch lenses.
- The type of assembly was significant at night and nearly not significant
(p-value = 0.0565) during the day. This indicates that the effects of the beacons/LEDs on reading the message on the sign are more influential during nighttime conditions, an expected finding. - Based on the sign legibility results, the suggested alternative beacon arrangement for testing in the on-road portion is the C-B12 assembly; the primary beacon assembly is the R-B assembly.
Observations Regarding Legibility Distance
The presence of a yellow flashing beacon communicates warning to a driver. Even if the flashing beacon limits the ability to read a sign, its presence can warn drivers to take additional care at the location. Unfortunately, the extensive and continuous use of the flashing yellow beacon on U.S. highways may not effectively communicate to drivers the needed action of slowing down or searching for a potential roadway entry. The use of a specific flash pattern, however, could offset some of these concerns. The profession should investigate the message that different styles of blinking lights (e.g., rapid flash versus uniform flash, etc.) communicate to drivers.
An objective of this study was to determine whether the detection of objects located beyond an assembly was affected by the shape, size, and/or placement of the yellow RRFBs/LEDs. This analysis is separated into daytime and nighttime because of large differences in detection distance between day and night and to determine whether variables were affecting object detection distances differently in day and night conditions. Each set was analyzed using LMMs. A number of different evaluations were conducted to focus on different elements of the study, such as day/night, different groups to account for sign face, or upstream conditions. The following variables were considered:
- Age (young or old).
- Object type (box, trash can, or pedestrian).
When focusing on characteristics of the study assemblies, the following variables were considered:
- Previous assembly (C-A12, C-B12, C-B8, C-V12, R-B, R-A, or LED). Preliminary results demonstrated that the WO-B device had too many unique qualities and should not be included in the evaluation. Those qualities included having only one object—the box—that followed the sign.
- Previous sign family (Ped X-ing, common signs, and uncommon signs).
When evaluating the effects of all upstream conditions, the following three new groups were created:
- Signs w/Bea-LED (C-A12, C-B12, C-B8, C-V12, R-B, R-A, or LED).
- Signs without Bea-LED (included the distractor signs and the WO-B assembly).
- Turn (represents the situation when the object was not located beyond a sign, i.e., the object was located alongside the road after the driver had just made a turn on the course).
To provide an appreciation of the range of object detection distance considered, table 113 lists the average and standard deviation of the object detection distance for the pedestrian, Table 114 is similar data for the trash can, and table 115 contains data for the small box.
Figure 62 shows the box plots for the data for the daytime while figure 63 shows the nighttime box plots. The limits of the box plots are at 25th and 75th percentiles. The whiskers were drawn at 1.5 times the interquartile range, and the widths were drawn proportional to the square root of the number of observations.
Table 113. Object detection distance for the pedestrian by upstream device and sign face.
Upstream devicea | Day | Night | ||||
Countb |
DD (ft) |
SD (ft) |
Count |
DD (ft) |
SD (ft) | |
A: C-A12-Trail Crossing | 16 | 962 | 617 | 11 | 54 | 33 |
A: C-A12-Wheelchair | 11 | 668 | 414 | 5 | 181 | 180 |
A: C-B12-Bike | 17 | 710 | 396 | 15 | 195 | 89 |
A: C-B12-Ped X-ing | 8 | 1,361 | 502 | 9 | 164 | 89 |
A: C-B8-Deer | 11 | 1,020 | 472 | 9 | 76 | 44 |
A: C-B8-Ped X-ing | 16 | 790 | 349 | 10 | 149 | 69 |
A: C-V12-Offset Intersection | 8 | 1,087 | 986 | 6 | 84 | 51 |
A: C-V12-Ped X-ing | 8 | 554 | 185 | 9 | 154 | 110 |
A: R-A-Ped X-ing | 8 | 1,242 | 527 | 11 | 177 | 119 |
A: R-A-Playground | 8 | 1,066 | 400 | 3 | 99 | 39 |
A: R-B-Bike & Ped | 19 | 980 | 788 | 18 | 82 | 75 |
A: R-B-Ped X-ing | 8 | 479 | 204 | 5 | 75 | 12 |
D: Always Animal | 34 | 1,228 | 438 | 30 | 98 | 105 |
D: Energy Supper | 0 | 0 | NAc | 1 | 102 | NAc |
D: Enough Silent | 35 | 664 | 312 | 24 | 89 | 55 |
Total Count/Average Distance | 207 | 911 | 539 | 166 | 116 | 93 |
aA: = assembly (see figure 23 to figure 38), D: = distractor sign (see table 96).
bCount = number of participants, DD = average detection distance (ft), SD = standard deviation detection distance (ft).
cNA = not applicable, two or more points are required for a standard deviation.
Table 114. Object detection distance for the trash can by upstream device and sign face.
Upstream devicea | Day | Night | ||||
Countb |
DD (ft) |
SD (ft) |
Count |
DD (ft) |
SD (ft) | |
A: C-A12-Trail Crossing | 9 | 722 | 215 | 6 | 135 | 67 |
A: C-A12-Wheelchair | 7 | 992 | 404 | 7 | 53 | 18 |
A: C-B12-Ped X-ing | 8 | 684 | 387 | 6 | 102 | 21 |
A: C-B8-Deer | 8 | 823 | 249 | 5 | 79 | 55 |
A: C-B8-Ped X-ing | 10 | 1,302 | 964 | 9 | 149 | 209 |
A: C-V12-Ped X-ing | 8 | 1,031 | 427 | 5 | 140 | 114 |
A: LEDs-Ped X-ing | 27 | 617 | 242 | 21 | 147 | 115 |
A: R-A-Ped X-ing | 7 | 1,341 | 978 | 5 | 155 | 92 |
A: R-B-Ped X-ing | 8 | 591 | 453 | 11 | 117 | 192 |
D: Speed Limit F4 | 31 | 657 | 336 | 22 | 75 | 45 |
D: Speed Limit H8 | 34 | 949 | 154 | 30 | 187 | 91 |
D: Stop Ahead (symbol) | 32 | 763 | 185 | 29 | 174 | 81 |
Turn | 69 | 650 | 109 | 65 | 276 | 137 |
Total Count/Average Distance | 258 | 773 | 378 | 221 | 179 | 133 |
aA: = assembly (see figure 23 to figure 38), D: = distractor sign (see table 96).
bCount = number of participants, DD = average detection distance (ft), SD = standard deviation detection distance (ft).
Table 115. Object detection distance for the box by upstream device and sign face.
Upstream devicea | Day | Night | ||||
Countb |
DD (ft) |
SD (ft) |
Count |
DD (ft) |
SD (ft) | |
A: C-A12-Trail Crossing | 0 | 0 | NAc | 1 | 100 | NAc |
A: C-A12-Wheelchair | 12 | 359 | 153 | 15 | 153 | 84 |
A: C-B12-Bike | 5 | 228 | 52 | 6 | 110 | 22 |
A: C-B12-Ped X-ing | 6 | 685 | 380 | 6 | 160 | 112 |
A: C-B8-Deer | 6 | 518 | 248 | 12 | 188 | 234 |
A: C-B8-Ped X-ing | 0 | 0 | NAc | 1 | 137 | NAc |
A: C-V12-Offset Intersection | 11 | 459 | 400 | 7 | 112 | 112 |
A: C-V12-Ped X-ing | 8 | 233 | 176 | 7 | 190 | 102 |
A: R-A-Ped X-ing | 9 | 233 | 216 | 8 | 337 | 293 |
A: R-A-See Saw | 9 | 294 | 143 | 13 | 117 | 100 |
A: R-B-Ped X-ing | 14 | 311 | 231 | 13 | 132 | 64 |
A: WO-B-T-Intersection | 27 | 200 | 170 | 33 | 145 | 62 |
D: Rough Road | 24 | 237 | 174 | 27 | 138 | 85 |
D: Speed Limit 42 | 12 | 309 | 141 | 23 | 150 | 92 |
D: Speed Limit 45 | 26 | 293 | 164 | 27 | 209 | 94 |
Turn | 56 | 235 | 139 | 81 | 166 | 84 |
Total Count/Average Distance | 225 | 286 | 210 | 280 | 162 | 110 |
aA: = assembly (see figure 23 to figure 38), D: = distractor sign (see table 96).
bCount = number of participants, DD = average detection distance (ft), SD = standard deviation detection distance (ft).
cNA = not applicable, two or more points are required for a standard deviation.
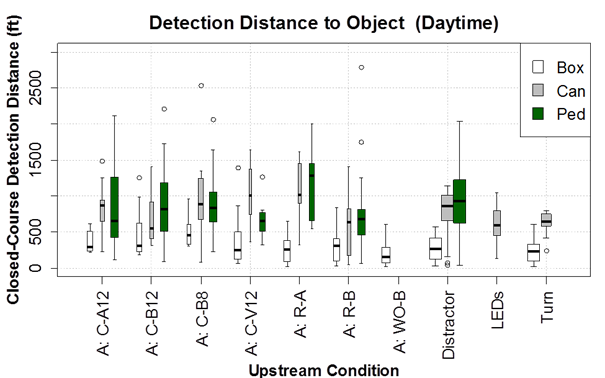
Figure 62. Graph. Box plot of daytime object detection distance by upstream condition.
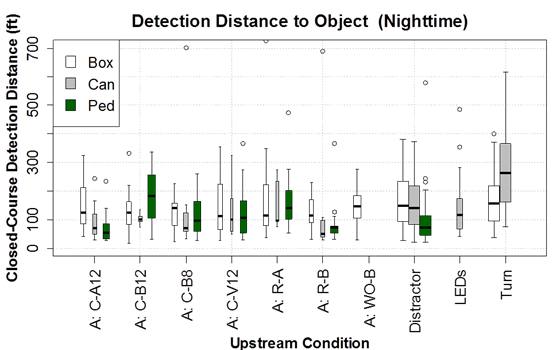
Figure 63. Graph. Box plot of nighttime object detection distance by upstream condition.
Daytime Object Detection Distance
Table 116 shows the LMM, and table 117 shows the ANOVA for daytime object detection distance with upstream conditions (i.e., this evaluation includes objects located behind distractor signs and after turns). All variables considered were significant: type of object (box, trash can, or pedestrian), age group (young or old), and upstream conditions. Finding upstream conditions significant indicates that the presence of a sign assembly and the characteristics of that sign assembly affect a participant’s ability to detect an object.
Table 116. Model for daytime object detection distance considering upstream condition.a
Variable |
Value |
Std. error |
DF |
t-value |
p-value |
Reference Levelb | 485.3553 |
35.77571 |
418 |
13.566617 |
0.0000 |
Object: box | -287.9107 |
33.86529 |
418 |
-8.501646 |
0.0000 |
Object: can | -68.0271 |
39.98690 |
418 |
-1.701234 |
0.0896 |
O.Y: young | 317.8674 |
57.58077 |
33 |
5.520374 |
0.0000 |
Upstream2: Distractor | -45.9384 |
22.90688 |
418 |
-2.005440 |
0.0456 |
Upstream2: Turn | -119.9760 |
34.51648 |
418 |
-3.475905 |
0.0006 |
Object: box X O.Y: young | -254.9557 |
54.31000 |
418 |
-4.694452 |
0.0000 |
Object: can X O.Y: young | -35.7910 |
72.27445 |
418 |
-0.495210 |
0.6207 |
aNotes:
- Linear mixed-effects model fit by REML.
- Fixed effects: Adj_Dis ~ Object * O.Y + Upstream2.
- Object = pedestrian.
- O.Y. (age: old or young) = older driver.
- Upstream2 = assembly.
Table 117. ANOVA results using upstream conditions daytime object detection distance.
Variable |
numDF |
denDF |
F-value |
p-value |
(Intercept) | 1 |
418 |
276.53328 |
< .0001 |
Object | 2 |
418 |
149.20799 |
< .0001 |
O.Y | 1 |
33 |
16.42528 |
0.0003 |
Upstream 2 | 2 |
418 |
5.64380 |
0.0038 |
Object X O.Y. | 2 |
418 |
13.87962 |
< .0001 |
Table 118 shows the results when focusing on objects near study assemblies (i.e., this evaluation does not include objects located behind the distractor signs or after turns). Table 119 shows the ANOVA results. These evaluations found that object type, age group, and previous sign assembly affect daytime object detection distance.
Table 118. Model for daytime object detection distance focusing on upstream assembly.a
Variable |
Value |
Std. error |
DF |
t-value |
p-value |
Reference Levelb | 404.6981 |
56.24747 |
220 |
7.194955 |
0.0000 |
Object: box | -323.8748 |
50.61636 |
220 |
-6.398619 |
0.0000 |
Object: can | -23.9229 |
53.96064 |
220 |
-0.443339 |
0.6580 |
O. Y: young | 296.6900 |
71.83064 |
33 |
4.130410 |
0.0002 |
Prev. Assembly: C-A12 | 133.9378 |
62.79891 |
220 |
2.132804 |
0.0340 |
Prev. Assembly: C-B12 | 154.7027 |
54.44614 |
220 |
2.841389 |
0.0049 |
Prev. Assembly: C-B8 | 166.9417 |
62.84257 |
220 |
2.656507 |
0.0085 |
Prev. Assembly: C-V12 | 77.9409 |
52.84642 |
220 |
1.474857 |
0.1417 |
Prev. Assembly: R-A | 156.6303 |
51.24030 |
220 |
3.056779 |
0.0025 |
Prev. Assembly: LEDs | -25.3392 |
72.64733 |
220 |
-0.348798 |
0.7276 |
Sign.Fam: Other Common | 120.6914 |
61.33491 |
220 |
1.967744 |
0.0504 |
Sign.Fam: Uncommon | -28.8649 |
49.59985 |
220 |
-0.581956 |
0.5612 |
Object: box X O.Y: young | -197.6446 |
70.31444 |
220 |
-2.810868 |
0.0054 |
Object:can X O.Y: young | 64.3989 |
84.82628 |
220 |
0.759186 |
0.4486 |
aNotes:
- Linear mixed-effects model fit by REML.
- Fixed effects: Adj_Dis ~ Object * O.Y + Prev.Assembly + Sign.Fam.
- Object = pedestrian.
- O.Y. (age: old or young) = older driver.
- Prev. Assembly = R-B.
- Sign.Fam (sign family) = common signs.
Table 119. ANOVAresults for daytime detection distance focusing on upstream assembly.
Variable |
numDF |
denDF |
F-value |
p-value |
(Intercept) | 1 |
220 |
254.85467 |
< .0001 |
Object | 2 |
220 |
77.19983 |
< .0001 |
O.Y | 1 |
33 |
14.81887 |
0.0005 |
Prev.Assembly | 6 |
220 |
4.03653 |
0.0007 |
Sign.Fam | 2 |
220 |
2.62041 |
0.0750 |
Object X O.Y | 2 |
220 |
6.56765 |
0.0017 |
Because the interaction between age and object type was significant (as shown in table 118), the pair comparisons shown in table 120 include all the possible combinations of object type and age. As expected, detection distances are significantly shorter for detecting a box. Older drivers detected a pedestrian 324 ft upstream compared with seeing a box behind the assembly, and an additional 300 ft for a trash can. Similarly, young drivers recognized a pedestrian 522 ft earlier than a box, and a can 562 ft earlier than a box. There is not sufficient evidence to suggest the detection distance for pedestrians and trash cans differed, either among old drivers (p-value of 0.997) or among young drivers (p-value of 0.993). However, detection distances by older drivers were significantly shorter compared with younger drivers, except when detecting boxes. Young drivers detected a box further upstream of the object compared with older drivers; however, the finding was not statistically significant. Young drivers outperformed old drivers by 300 ft when detecting a pedestrian and by 361 ft when detecting a trash can.
Table 120. Daytime object detection distance, multiple comparisons by object type and age group.
Linear hypothesesa |
Estimate |
Std. error |
z-value |
Pr(>|z|) b |
Box (Old) - Ped (Old) = 0 | -323.87 |
50.62 |
-6.399 |
< 0.001*** |
Can (Old) - Ped (Old) = 0 | -23.92 |
53.96 |
-0.443 |
0.997 |
Can (Old) - Box (Old) = 0 | 299.95 |
59.24 |
5.064 |
< 0.001*** |
Box (Young) - Ped (Young) = 0 | -521.52 |
55.00 |
-9.482 |
< 0.001*** |
Can (Young) - Ped (Young) = 0 | 40.48 |
75.61 |
0.535 |
0.993 |
Can (Young) - Box (Young) = 0 | 562.00 |
69.61 |
8.074 |
< 0.001*** |
Young (Ped) - Old (Ped) = 0 | 296.69 |
71.83 |
4.130 |
< 0.001*** |
Young (Box) - Old (Box) = 0 | 99.05 |
67.69 |
1.463 |
0.624 |
Young (Can) - Old (Can) = 0 | 361.09 |
82.18 |
4.394 |
< 0.001*** |
a Simultaneous tests for general linear hypotheses.
b Significance values are as follows: p < 0.10; * p < 0.05; ** p < 0.01; and *** p < 0.001. Adjusted p-values reported single-step method.
For daytime object detection distance, the legend on the sign was not significant. As shown in table 121, no significant differences were identified in the comparison of the Ped X-ing sign face with other common signs or to uncommon signs.
Table 121. Daytime object detection distance, multiple comparisons by sign family.
Linear hypothesesa |
Estimate |
Std. error |
z-value |
Pr(>|z|) b |
(Ped X-ing) – (Other Common) = 0 | -120.69 |
61.33 |
-1.968 |
0.112 |
(Ped X-ing) – (Uncommon) = 0 | 28.86 |
49.60 |
0.582 |
0.820 |
(Uncommon) – (Other Common) = 0 | -149.56 |
79.94 |
-1.871 |
0.138 |
aSimultaneous tests for general linear hypotheses.
bSignificance values are as follows: p < 0.10; * p < 0.05; ** p < 0.01; and *** p < 0.001. Adjusted p-values reported single-step method.
The characteristics of the beacons/LEDs did influence some of the object detection distances as shown in table 122 and table 123. Objects were detected at a greater distance upstream when located beyond the C-B12, C-B8, and R-A assemblies compared with R-B. During daytime conditions, the object detection distance was between 155 to 167 ft longer for the C-B12, C-B8, and R-A assemblies compared with the R-B assembly.
Table 122. Daytime object detection distance, multiple comparisons to reference assembly by assembly type.
Linear Hypothesesa |
Estimate |
Std. error |
z-value |
Pr(>|z|) b |
(C-A12) - (R-B) = 0 | 133.94 |
62.80 |
2.133 |
0.1656 |
(C-B12) - ( R-B ) = 0 | 154.70 |
54.45 |
2.841 |
0.0253* |
(C-B8) - (R-B) = 0 | 166.94 |
62.84 |
2.657 |
0.0435* |
(C-V12) - (R-B) = 0 | 77.94 |
52.85 |
1.475 |
0.5467 |
(R-A) - (R-B) = 0 | 156.63 |
51.24 |
3.057 |
0.0129* |
(LEDs) - (R-B) = 0 | -25.34 |
72.65 |
-0.349 |
0.9993 |
aSimultaneous tests for general linear hypotheses.
bSignificance values are as follows: p < 0.10; * p < 0.05; ** p < 0.01; and *** p < 0.001. Adjusted p-values reported single-step method.
Table 123. Daytime object detection distance, other multiple comparisons by assembly type.
Linear Hypothesesa |
Estimate |
Std. error |
z-value |
Pr(>|z|) b |
(C-B12) - (C-A12) = 0 | 20.765 |
58.518 |
0.355 |
0.999 |
(C-B8 ) - (C-A12) = 0 | 33.004 |
81.692 |
0.404 |
0.999 |
(C-V12) - (C-A12) = 0 | -55.997 |
75.149 |
-0.745 |
0.975 |
(R-A) - (C-A12) = 0 | 22.692 |
62.378 |
0.364 |
0.999 |
(LEDs ) - (C-A12) = 0 | -159.277 |
83.685 |
-1.903 |
0.389 |
(C-B8 ) - (C-B12) = 0 | 12.239 |
72.839 |
0.168 |
1.000 |
(C-V12) - (C-B12) = 0 | -76.762 |
66.221 |
-1.159 |
0.850 |
(R-A) - (C-B12) = 0 | 1.928 |
57.779 |
0.033 |
1.000 |
(LEDs) - (C-B12) = 0 | -180.042 |
79.316 |
-2.270 |
0.199 |
(C-V12) - (C-B8) = 0 | -89.001 |
60.333 |
-1.475 |
0.670 |
(R-A) - (C-B8) = 0 | -10.311 |
73.408 |
-0.140 |
1.000 |
(LEDs) - (C-B8) = 0 | -192.281 |
82.307 |
-2.336 |
0.173 |
(R-A) - (C-V12) = 0 | 78.689 |
61.727 |
1.275 |
0.791 |
(LEDs) - (C-V12) = 0 | -103.280 |
81.212 |
-1.272 |
0.792 |
(LEDs) - (R-A) = 0 | -181.969 |
78.915 |
-2.306 |
0.184 |
aSimultaneous tests for general linear hypotheses.
bAdjusted p-values reported – single-step method.
Table 124 shows the comparisons regarding beacon shape and placement with none being significant. The daytime distance to detect an object located beyond a study assembly was similar for the circular and rectangular beacon shape. The distances were also similar when the beacons were located above the sign compared with when the beacons were located below the sign. The comparison of the 12-inch beacon to the 8-inch beacon is contained in table 123 with the result being no significant difference in daytime detection distance to the object.
Table 124. Daytime object detection distance for beacon placement.
Linear Hypothesesa |
Estimate |
Std. error |
z-value |
Pr(>|z|) b |
(Circular) - (Rectangular) = 0 | 73.54 |
39.30 |
1.871 |
0.142 |
(Above) - (Below) [All] = 0 | 38.08 |
43.61 |
0.873 |
0.679 |
(Above) - (Below) [Without B8] = 0 | 67.93 |
40.24 |
1.688 |
0.206 |
aSimultaneous tests for general linear hypotheses.
bAdjusted p-values reported – single-step method.
Nighttime Object Detection Distance
Table 125 and table 126 show the results regarding nighttime object detection distance when considering upstream conditions (i.e., this evaluation includes objects located behind distractor signs and after turns). Unlike daytime conditions, there was not sufficient evidence to suggest a difference in detection distance between younger and older drivers. Similar to daytime conditions, detection distance differences exist for the object type and for upstream conditions. Even though they were statistically significant, the detection distance differences at night were smaller and may not be of practical meaningfulness.
The box was detected 23 ft prior to the pedestrian (statistically significant), and the trash can was detected 9 ft prior to the pedestrian (not statistically significant). A significant difference was not identified between the detection distance for the objects beyond the distractor signs and the objects beyond the signs with beacons or LEDs. A difference was identified for the distance to the object after a turn (i.e., when no sign is present) and objects following a sign with beacons or LEDs. When participants did not have to identify a sign’s presence and to read the sign face, they were able to detect objects an additional 31 ft upstream.
Table 125. Model for nighttime object detection distance considering upstream condition.a
Variable |
Value |
Std. error |
DF |
t-value |
p-value |
Reference Levelb | 63.08422 |
6.492316 |
505 |
9.716751 |
0.0000 |
Object: box | 22.53531 |
5.550685 |
505 |
4.059915 |
0.0001 |
Object: can | 9.47397 |
5.274080 |
505 |
1.796327 |
0.0730 |
O.Y: young | 0.84664 |
7.654252 |
34 |
0.110610 |
0.9126 |
Upstream2: Distractor | -2.70914 |
4.609055 |
505 |
-0.587787 |
0.5569 |
Upstream2: Turn | 31.02125 |
8.375902 |
505 |
3.703631 |
0.0002 |
aNotes:
- Linear mixed-effects model fit by REML.
- Fixed effects: Adj_Dis ~ Object + O.Y + Upstream2.
- Object = pedestrian.
- O.Y. (age: old or young) = older driver.
- Upstream2 = assembly.
Table 126. ANOVA results for nighttime object detection distance considering upstream. condition.
Variable |
numDF |
denDF |
F-value |
p-value |
(Intercept) | 1 |
505 |
388.7771 |
< .0001 |
Object | 2 |
505 |
15.4687 |
< .0001 |
O.Y | 1 |
34 |
0.0004 |
0.9832 |
Upstream2 | 2 |
505 |
8.1229 |
0.0003 |
Table 127 and table 128 show the results when focusing on the type of beacon/LED of the previous assembly. The base condition is older participant, with the object being a pedestrian located beyond an R-B with a Ped X-ing sign. As shown in table 127 and confirmed with the simultaneous tests shown in table 129, age was not a significant variable regarding object detection distance at night. The results in table 127 imply significant differences in detection distance by type of object; however, the pair comparisons in table 130 demonstrate that the only significant difference was between the trash can and the box with an estimated distance difference of 24 ft.
Table 127. Model for nighttime object detection distance considering upstream assembly.a
Variable |
Value |
Std. error |
DF |
t-value |
p-value |
Reference Levelb | 64.68411 |
10.206352 |
194 |
6.337632 |
0.0000 |
Object: box | 10.54916 |
7.423142 |
194 |
1.421118 |
0.1569 |
Object: can | -13.00810 |
7.951071 |
194 |
-1.636019 |
0.1035 |
O.Y: young | 13.46431 |
9.203264 |
34 |
1.462993 |
0.1527 |
Prev.Assembly: C-A12 | 17.79469 |
10.677269 |
194 |
1.666596 |
0.0972 |
Prev.Assembly: C-B12 | 35.22210 |
11.293281 |
194 |
3.118854 |
0.0021 |
Prev.Assembly: C-B8 | 23.19978 |
12.498281 |
194 |
1.856238 |
0.0649 |
Prev.Assembly: C-V12 | 22.16982 |
12.481922 |
194 |
1.776154 |
0.0773 |
Prev.Assembly: R-A | 23.26325 |
11.247088 |
194 |
2.068380 |
0.0399 |
Prev.Assembly: LEDs | 24.52451 |
15.412787 |
194 |
1.591179 |
0.1132 |
Sign.Fam: Other Common | -31.77985 |
11.438550 |
194 |
-2.778311 |
0.0060 |
Sign.Fam: Uncommon | -22.72245 |
9.561413 |
194 |
-2.376474 |
0.0185 |
aNotes:
- Linear mixed-effects model fit by REML.
- Fixed effects: Adj_Dis ~ Object + O.Y + Prev.Assembly + Sign.Fam.
- Object = pedestrian.
- O.Y. (age: old or young) = older driver.
- Prev.Assembly = R-B.
- Sign.Fam (sign family) = common signs.
Table 128. ANOVA results using for nighttime object detection distance considering upstream assembly.
Variable |
numDF |
denDF |
F-value |
p-value |
(Intercept) |
1 |
194 |
257.49711 |
< .0001 |
Object |
2 |
194 |
3.61435 |
0.0288 |
O.Y |
1 |
34 |
1.47261 |
0.2333 |
Prev.Assembly |
6 |
194 |
2.46570 |
0.0254 |
Sign.Fam |
2 |
194 |
6.27471 |
0.0023 |
Table 129. Nighttime object detection distance, multiple comparisons by age group.
Linear hypothesesa |
Estimate |
Std. error |
z value |
Pr(>|z|) b |
Young - Old = 0 |
13.464 |
9.203 |
1.463 |
0.143 |
aSimultaneous tests for general linear hypotheses.
bAdjusted p values reported single-step method.
Table 130. Nighttime object detection distance, multiple comparisons by object type.
Linear hypothesesa |
Estimate |
Std. error |
z value |
Pr(>|z|) b |
(Ped X-ing) - (Other Common) = 0 |
31.780 |
11.439 |
2.778 |
0.0143* |
(Ped X-ing) - (Uncommon) = 0 |
22.722 |
9.561 |
2.376 |
0.0437* |
(Uncommon) - (Other Common) = 0 |
9.057 |
14.416 |
0.628 |
0.7976 |
aSimultaneous tests for general linear hypotheses.
bSignificance values are as follows: p < 0.10; * p < 0.05; ** p < 0.01; and *** p < 0.001. Adjusted p values reported single-step method.
Detecting the object occurred at slightly greater distances when beyond the signs showing Ped X-ing compared with uncommon signs (23 ft) or other common signs (32 ft) as shown in table 131.
Table 131. Nighttime object detection distance, multiple comparisons by sign family.
Linear hypothesesa |
Estimate |
Std. error |
z-value |
Pr(>|z|) b |
(Ped X-ing) - (Other Common) = 0 |
31.780 |
11.439 |
2.778 |
0.0143* |
(Ped X-ing) - (Uncommon) = 0 |
22.722 |
9.561 |
2.376 |
0.0437* |
(Uncommon) - (Other Common) = 0 |
9.057 |
14.416 |
0.628 |
0.7976 |
aSimultaneous tests for general linear hypotheses.
bSignificance values are as follows: p < 0.10; * p < 0.05; ** p < 0.01; and *** p < 0.001. Adjusted p values reported single-step method.
Table 132 and table 133 are pair comparisons between different beacon/LED types. Only one paired comparison had a statistically significant result (C-B12 to R-B). In all other cases, the detection distance to the object was not significantly different among the various beacon/LED assemblies. During nighttime conditions, objects were detected 35 ft farther upstream with the C‑B12 than with the R-B. The objects were also detected at a greater distance upstream during the daytime with C-B12 compared with R-B (155 ft).
Table 134 shows the comparisons regarding beacon shape and placement with none being significant. The distance to detect an object located beyond a study assembly was similar for the circular and rectangular beacon shape. The distances were also similar when the beacons were located above the sign compared with when the beacons were located below the sign. The comparison of the 12-inch beacon to the 8-inch beacon is contained in table 133 with the result being no significant difference in detection distance to the object.
Table 132. Nighttime object detection distance, multiple comparisons to reference assembly by assembly type.
Linear hypothesesa |
Estimate |
Std. error |
z-value |
Pr(>|z|) b |
(C-A12) - (R-B) = 0 |
17.79 |
10.68 |
1.667 |
0.4148 |
(C-B12) - (R-B) = 0 |
35.22 |
11.29 |
3.119 |
0.0106* |
(C-B8 ) - (R-B) = 0 |
23.20 |
12.50 |
1.856 |
0.2969 |
(C-V12) - (R-B) = 0 |
22.17 |
12.48 |
1.776 |
0.3440 |
(R-A) - (R-B) = 0 |
23.26 |
11.25 |
2.068 |
0.1928 |
(LEDs) - (R-B) = 0 |
24.52 |
15.41 |
1.591 |
0.4672 |
aSimultaneous tests for general linear hypotheses.
bSignificance values are as follows: p < 0.10; * p < 0.05; ** p < 0.01; and *** p < 0.001. Adjusted p values reported single-step method.
Table 133. Nighttime object detection distance, other multiple comparisons by assembly type.
Linear hypothesesa |
Estimate |
Std. error |
z-value |
Pr(>|z|) b |
(C-B12) - (C-A12) = 0 |
17.42741 |
12.66434 |
1.376 |
0.732 |
(C-B8 ) - (C-A12) = 0 |
5.40509 |
15.39298 |
0.351 |
0.999 |
(C-V12) - (C-A12) = 0 |
4.37513 |
15.21513 |
0.288 |
1.000 |
(R-A) - (C-A12) = 0 |
5.46856 |
12.06913 |
0.453 |
0.997 |
(LEDs) - (C-A12) = 0 |
6.72982 |
17.24016 |
0.390 |
0.999 |
(C-B8) - (C-B12) = 0 |
-12.02232 |
14.80255 |
-0.812 |
0.964 |
(C-V12) - (C-B12) = 0 |
-13.05228 |
14.21682 |
-0.918 |
0.939 |
(R-A) - (C-B12) = 0 |
-11.95885 |
13.09877 |
-0.913 |
0.940 |
(LEDs ) - (C-B12) = 0 |
-10.69759 |
17.28384 |
-0.619 |
0.989 |
(C-V12) - (C-B8) = 0 |
-1.02996 |
11.39493 |
-0.090 |
1.000 |
(R-A) - (C-B8) = 0 |
0.06347 |
15.31347 |
0.004 |
1.000 |
(LEDs) - (C-B8) = 0 |
1.32473 |
16.81555 |
0.079 |
1.000 |
( R-A) - (C-V12) = 0 |
1.09343 |
14.83082 |
0.074 |
1.000 |
(LEDs) - (C-V12) = 0 |
2.35469 |
17.25943 |
0.136 |
1.000 |
(LEDs) - (R-A) = 0 |
1.26126 |
17.64379 |
0.071 |
1.000 |
aSimultaneous tests for general linear hypotheses.
bSignificance values are as follows: p < 0.10; * p < 0.05; ** p < 0.01; and *** p < 0.001. Adjusted p values reported single-step method.
Table 134. Nighttime object detection distance for beacon placement.
Linear hypothesesa |
Estimate |
Std. error |
z-value |
Pr(>|z|) |
(Circular) - (Rectangular) = 0 | 13.773 |
7.624 |
1.807 |
0.156 |
(Above) - (Below) [All] = 0 | 1.057 |
9.038 |
0.117 |
0.998 |
(Above) - (Below) [Without B8] = 0 | 2.918 |
8.650 |
0.337 |
0.960 |
aSimultaneous tests for general linear hypotheses.
Key Findings Regarding Object Detection Distance
For the analysis focusing on object detection distance, which is the distance between the object and the participant when the participant says “ped”, “can,” or “box,” results indicate the following:
- As expected, there is a significant difference between daytime and nighttime object detection distance. As an example, the daytime detection distance to a pedestrian had a mean of 911 ft and a standard deviation of 539ft. In contrast, during the night, the detection distance to a pedestrian had a mean of only 116 and a standard deviation of 93ft.
- Similar to legibility distance, there was a statistically significant difference owing to age during the daytime; surprisingly, the same finding did not occur at night. The nighttime condition itself seems to impede detection to a point that the effects of several variables are too small to detect in the experiment.
- The detection distance to a pedestrian or to a trash can was similar for both daytime and nighttime conditions. While the pedestrians were a few feet taller than the can cans and had a similar width, they were much larger than the small box. As expected, the detection distance to a box compared with the pedestrian was shorter during the daytime by 324 ft (older drivers) or 522 ft (younger drivers). Surprisingly, the nighttime detection distance to the box was slightly longer (by about 24 ft) compared with the can.
- Certain assemblies were associated with shorter object detection distances. For daytime conditions, the detection distance to an object was shorter for the R-B than with the C‑B12, C-B8, or the R-A (statistically significant). During the nighttime, the detection distance to an object was statistically significantly shorter with the R-B than with the C‑B12. These findings indicate that characteristics of the R-B, such as the light intensity or the location of the beacon beneath the sign, may negatively affect driver’s ability to see an object beyond the assembly.
- For both daytime and nighttime conditions, similar object detection distance results regardless of the shape of the beacons (circular or rectangular) or the placement of the beacons (above or below).
- Based on the above results and similar to legibility distance recommendation, the suggested alternative for testing in the on-road study is the C-B12 assembly.
Accuracy of Detecting an Object
The previous section discussed the detection distance to an object that was identified. The analysis did not consider when a participant missed the object; in other words, situations where the participant did not say “box,” “ped,” or “can” when that object was present. This section identifies factors that contribute to drivers missing an object and examines the influence of the preceding assembly on whether an object was missed.
The evaluation of the accuracy of detecting an object considered whether the presence of a sign affected a participant missing an object. Similar to the evaluation on object detection distance, for this evaluation, the following variables were considered:
- Time (day or night).
- Age (young or old).
- Object type (box, trash can, or pedestrian).
- Upstream2 (grouped by signs with Beacon-LED, distractor signs, and turn. The turn group represents the situation when the object was not located beyond a sign. This variable does not include the WO-B device because it only had one object—the box—that followed the sign.).
The evaluation then continued with focusing on those situations when an object followed a sign that had some type of beacon or LED. For this evaluation, the following variables were considered:
- Time (day or night).
- Age (young or old).
- Object type (box, can, or ped).
- Previous device (C-A12, C-B12, C-B8, C-V12, R-B, R-A, or LED).
- Previous sign family (Ped X-ing, common signs, and uncommon signs).
To provide an appreciation of the results, table 135 and table 136 show the frequency (count) of objects detected and missed along with the percent missed. The results in table 135 show differences in percent missed by object type, by upstream condition, and by light level. More objects were missed during nighttime conditions: 21 percent of the boxes, 21 percent of the trash cans, and 21 percent of the pedestrians. Said in another way, about one in five objects were missed at night. During the daytime, fewer pedestrians and trash cans were missed; however, more boxes were missed. Table 136 shows similar data with a focus on age of the participant. Older drivers missed more of the objects (26 percent overall) compared with younger drivers (12percent overall). Because other factors are known to affect the results of detection distances, the following statistical evaluation indicates whether these preliminary observations are statistically significant.
Table 135. Object percent missed by previous device group, object type, and light condition.
Action | Previous device group | Day | Night | Total | ||||||
Box | Can | Ped | Total | Box | Can | Ped | Total | |||
Miss | Sign with Beacon-LED | 27 | 11 | 2 | 40 | 19 | 28 | 29 | 76 | 116 |
Distractor | 41 | 7 | 0 | 48 | 30 | 23 | 15 | 68 | 116 | |
Turn | 44 | 1 | 0 | 45 | 24 | 7 | 0 | 31 | 76 | |
WO-B | 7 | 0 | 0 | 7 | 3 | 0 | 0 | 3 | 10 | |
Total | 119 | 19 | 2 | 140 | 76 | 58 | 44 | 178 | 318 | |
Saw | Sign with Beacon-LED | 80 | 92 | 138 | 310 | 89 | 75 | 111 | 275 | 585 |
Distractor | 62 | 97 | 69 | 228 | 77 | 81 | 55 | 213 | 441 | |
Turn | 56 | 69 | 0 | 125 | 81 | 65 | 0 | 146 | 271 | |
WO-B | 27 | 0 | 0 | 27 | 33 | 0 | 0 | 33 | 60 | |
Total | 225 | 258 | 207 | 690 | 280 | 221 | 166 | 667 | 1357 | |
Percent | Sign with Beacon-LED | 25% | 11% | 1% | 11% | 18% | 27% | 21% | 22% | 17% |
Distractor | 40% | 7% | 0% | 17% | 28% | 22% | 21% | 24% | 21% | |
Turn | 44% | 1% | NA | 26% | 23% | 10% | NA | 18% | 22% | |
WO-B | 21% | NA | NA | 21% | 8% | NA | NA | 8% | 14% | |
All | 35% | 7% | 1% | 17% | 21% | 21% | 21% | 21% | 19% |
Ped = Pedestrian.
NA = percentage not calculated because no events with those properties occurred.
Table 136. Object percent missed by age group, object type, and light condition.
Action | Age | Day | Night | Total | ||||||
Box | Can | Ped | Total | Box | Can | Ped | Total | |||
Miss | Old | 78 | 15 | 2 | 95 | 56 | 38 | 25 | 119 | 214 |
Young | 41 | 4 | 0 | 45 | 20 | 20 | 19 | 59 | 104 | |
Both | 119 | 19 | 2 | 140 | 76 | 58 | 44 | 178 | 318 | |
Saw | Old | 95 | 126 | 106 | 327 | 111 | 93 | 71 | 275 | 602 |
Young | 130 | 132 | 101 | 363 | 169 | 128 | 95 | 392 | 755 | |
Both | 225 | 258 | 207 | 690 | 280 | 221 | 166 | 667 | 1357 | |
Percent | Old | 45% | 11% | 2% | 23% | 34% | 29% | 26% | 30% | 26% |
Young | 24% | 3% | 0% | 11% | 11% | 14% | 17% | 13% | 12% | |
Both | 35% | 7% | 1% | 17% | 21% | 21% | 21% | 21% | 19% |
NA = percentage not calculated because no events with those properties occurred.
Because the data structure is the same for the percent miss and object detection distance variables, the blocking structure for this analysis was preserved (i.e., light condition/driver/lap number). The data were then split by daytime and nighttime conditions so that each set could be analyzed using Generalized Linear Mixed Effects Models (GLMM). These kinds of models are very similar to LMMs in that they treat random effects as blocking factors, and the fixed effects as parameter estimates. The key difference is that because the response variable is binary (1 if the object was missed, 0 otherwise), the estimation requires specifying a link function (logistic function in this case) as Generalized Linear Models also do. Weights were not applied in this case.
Daytime Object Detection Accuracy
Table 137 shows the results regarding detecting objects during the daytime when considering upstream conditions (i.e., this evaluation includes objects located behind distractor signs and after turns). The probability of detecting an object was statistically significantly different for the age groups (older drivers were more likely to miss the object) and object type (participants were more likely to miss a box or a trash can than the pedestrian). The upstream condition was not statistically significant. In other words, it did not matter whether the object was beyond a sign with beacons or LEDs or beyond one of the detector signs or not beyond a sign (i.e., following a turn). The probability of missing an object was similar regardless of the upstream condition.
Table 138 shows the results when focusing on the type of beacon/LED of the previous assembly. The base condition is older participant, with the object being a pedestrian located beyond an R-B with a Ped X-ing sign. Similar to the findings in table 137, age group and object type were significant. The findings in table 138 also show that the probability of missing an object is sensitive to the type of beacons/LED when compared with the base condition of R-B. R-A was the only previous assembly that was not significantly different.
Table 137. Model for daytime object detection accuracy considering upstream condition.a
Variables |
Value |
Std. error |
DF |
t-value |
p-value |
Reference Levelb | -5.065441 |
0.6706133 |
721 |
-7.553446 |
0.0000 |
O.Y: young | -1.040132 |
0.4196777 |
33 |
-2.478406 |
0.0185 |
Object: box | 4.621094 |
0.6284863 |
721 |
7.352736 |
0.0000 |
Object: can | 2.105317 |
0.6404133 |
721 |
3.287434 |
0.0011 |
Upstream2: Distractor | 0.450288 |
0.2384175 |
721 |
1.888652 |
0.0593 |
Upstream2: Turn | 0.303752 |
0.2534788 |
721 |
1.198334 |
0.2312 |
aNotes:
- Linear mixed-effects model fit by maximum likelihood.
- 2. Fixed effects: Miss ~ O.Y + Object + Upstream2.
- O.Y. (age: old or young) = older driver.
- Object = pedestrian.
- Upstream2 = assembly.
Simultaneous tests were conducted to determine whether differences exist for selected comparisons. Results are shown in table 138 to table 142.
The model results confirm a statistically significant difference by object type (see table 138), with both box and trash can being more likely to be missed than a pedestrian. In addition, the box was more likely to be missed than the trash can. These results are expected because the box is a much smaller target than either the trash can or the pedestrian. Young drivers were less likely to miss objects than old drivers as shown in table 139.
After controlling for other factors, there was no evidence of different probability of missing an object following the Ped X-ing and other common signs. Strong evidence is present for the odds of missing objects after uncommon signs as shown in table 140; the added challenge of reading and interpreting uncommon signs may have resulted in more drivers missing objects behind them.
Table 141 shows the results for the multiple assembly comparisons when each assembly is compared with the R-B. Table 142 provides the results between the different assemblies. The results indicate several pairs of devices that were associated with the participants being more likely to miss an object during the daytime. Recall, as shown in table 135, the object most likely missed was the small box.
Table 144 shows the results when examining the effects of beacon shape and placement. The shape of the beacons did not result in statistically significant differences; a similar probability of missing the object was present whether the beacons were circular or rectangular. If the beacons were located above or below the sign did matter; when beacons were above the sign, the participants were less likely to miss the object. The same results were found whether the below group included all beneath beacons (i.e., R-B, C-B12, and C-B8) or only those beacons beneath the sign that had a direct match with above beacons (i.e., R-B and C-B12 compared with R-A and C-A12).
The size of the circular beacon did not have an effect of the probability of missing an object. As shown in table 143, the difference between C-B8 and C-B12 was not statistically significant.
Table 138. Model for daytime object detection accuracy considering upstream assembly.a
Variables |
Value |
Std. error |
DF |
t-value |
p-value |
Reference Levelb | -31.036509 |
.429661 |
270 |
-9.049439 |
0.0000 |
O.Y: young | -7.604175 |
3.355508 |
33 |
-2.266177 |
0.0301 |
Object: box | 22.528656 |
2.060295 |
270 |
10.934673 |
0.0000 |
Object: can | 12.075817 |
1.600502 |
270 |
7.545019 |
0.0000 |
Prev.Assembly: C-A12 | -5.471284 |
1.604065 |
270 |
-3.410887 |
0.0007 |
Prev.Assembly: C-B12 | 3.308869 |
1.139095 |
270 |
2.904824 |
0.0040 |
Prev.Assembly: C-B8 | 7.405977 |
1.412711 |
270 |
5.242386 |
0.0000 |
Prev.Assembly: C-V12 | 1.985354 |
0.896321 |
270 |
2.215004 |
0.0276 |
Prev.Assembly: R-A | 1.363892 |
1.116743 |
270 |
1.221312 |
0.2230 |
Prev.Assembly: LEDs | 13.164725 |
1.781986 |
270 |
7.387669 |
0.0000 |
Sign.Fam: Other Common | -1.117426 |
1.433653 |
270 |
-0.779426 |
0.4364 |
Sign.Fam: Uncommon | 17.846180 |
1.817777 |
270 |
9.817586 |
0.0000 |
aNotes:
- Linear mixed-effects model fit by maximum likelihood.
- Fixed effects: Miss ~ O.Y + Object + Prev.Assembly + Sign.Fam.
- O.Y. = older driver.
- Object = pedestrian.
- Prev.Assembly = R-B.
- Sign.Fam = common.
Table 139. Daytime object detection accuracy, multiple comparisons by object type.
Linear hypothesesa | Estimate | Std. error | z-value | Pr(>|z|) b |
Box - Ped = 0 | 22.529 | 2.025 | 11.127 | < 1e-10*** |
Can - Ped = 0 | 12.076 | 1.573 | 7.678 | < 1e-10*** |
Can - Box = 0 | -10.453 | 1.385 | -7.545 | < 1e-10*** |
aSimultaneous tests for general linear hypotheses.
bSignificance values are as follows: p < 0.10; * p < 0.05; ** p < 0.01; and *** p < 0.001. Adjusted p values reported single-step method.
Table 140. Daytime object detection accuracy, multiple comparisons by age group.
>Linear hypothesesa | Estimate | Std. error | z-value | Pr(>|z|) b |
Young - Old = 0 | -7.604 | 3.297 | -2.306 | 0.0211 * |
aSimultaneous tests for general linear hypotheses.
bSignificance values are as follows: p < 0.10; * p < 0.05; ** p < 0.01; and *** p < 0.001. Adjusted p values reported single-step method.
Table 141. Daytime object detection accuracy, multiple comparisons by sign family.
Linear hypothesesa |
Estimate |
Std. error |
z-value |
Pr(>|z|) b |
(Ped X-ing) - (Other Common) = 0 | 1.117 |
1.409 |
0.793 |
0.681 |
(Ped X-ing) - (Uncommon) = 0 | -17.846 |
1.786 |
-9.990 |
< 1e-04*** |
(Uncommon) - (Other Common) = 0 | 18.964 |
2.498 |
7.591 |
< 1e-04*** |
a Simultaneous tests for general linear hypotheses.
b Significance values are as follows: p < 0.10; * p < 0.05; ** p < 0.01; and *** p < 0.001. Adjusted p values reported single-step method.
Table 142. Daytime object detection accuracy, multiple comparisons to reference assembly by assembly type.
Linear hypothesesa |
Estimate |
Std. error |
z-value |
Pr(>|z|) b |
(C-A12) - ( R-B ) = 0 | -5.4713 |
1.5763 |
-3.471 |
0.00277** |
(C-B12) - ( R-B ) = 0 | 3.3089 |
1.1194 |
2.956 |
0.01564 * |
(C-B8 ) - ( R-B ) = 0 | 7.4060 |
1.3883 |
5.335 |
< 0.001*** |
(C-V12) - ( R-B ) = 0 | 1.9854 |
0.8808 |
2.254 |
0.10620 |
( R-A ) - ( R-B ) = 0 | 1.3639 |
1.0974 |
1.243 |
0.63116 |
(LEDs) - (R-B ) = 0 | 13.1647 |
1.7512 |
7.518 |
< 0.001*** |
aSimultaneous tests for general linear hypotheses.
bSignificance values are as follows: p < 0.10; * p < 0.05; ** p < 0.01; and *** p < 0.001. Adjusted p values reported single-step method.
Table 143. Daytime object detection accuracy, other multiple comparisons by assembly type.
Linear hypothesesa |
Estimate |
Std. error |
z-value |
Pr(>|z|) b |
(C-B12) - (C-A12) = 0 | 8.7802 |
1.2159 |
7.221 |
< 0.001*** |
(C-B8 ) - (C-A12) = 0 | 12.8773 |
2.0258 |
6.356 |
< 0.001*** |
(C-V12) - (C-A12) = 0 | 7.4566 |
1.7470 |
4.268 |
< 0.001*** |
( R-A ) - (C-A12) = 0 | 6.8352 |
1.1431 |
5.980 |
< 0.001*** |
(LEDs) - (C-A12) = 0 | 18.6360 |
1.8148 |
10.269 |
< 0.001*** |
(C-B8 ) - (C-B12) = 0 | 4.0971 |
1.7388 |
2.356 |
0.14048 |
(C-V12) - (C-B12) = 0 | -1.3235 |
1.3524 |
-0.979 |
0.89861 |
( R-A ) - (C-B12) = 0 | -1.9450 |
0.5526 |
-3.520 |
0.00437** |
(LEDs ) - (C-B12) = 0 | 9.8559 |
1.5051 |
6.548 |
< 0.001*** |
(C-V12) - (C-B8 ) = 0 | -5.4206 |
1.1426 |
-4.744 |
< 0.001*** |
( R-A ) - (C-B8 ) = 0 | -6.0421 |
1.7680 |
-3.417 |
0.00664** |
(LEDs ) - (C-B8 ) = 0 | 5.7587 |
1.2991 |
4.433 |
< 0.001*** |
( R-A ) - (C-V12) = 0 | -0.6215 |
1.3740 |
-0.452 |
0.99638 |
(LEDs ) - (C-V12) = 0 | 11.1794 |
1.6652 |
6.714 |
< 0.001*** |
(LEDs ) - ( R-A ) = 0 | 11.8008 |
1.4812 |
7.967 |
< 0.001*** |
aSimultaneous tests for general linear hypotheses.
bSignificance values are as follows: p < 0.10; * p < 0.05; ** p < 0.01; and *** p < 0.001. Adjusted p values reported single-step method.
Table 144. Daytime object detection accuracy for beacon placement.
Linear hypothesesa |
Estimate |
Std. error |
z-value |
Pr(>|z|) b |
(Circular) - (Rectangular) = 0 | 1.0659 |
0.6903 |
1.544 |
0.257 |
(Above) - (Below) [All] = 0 | -5.6250 |
1.0494 |
-5.360 |
< 0.001*** |
(Above) - (Below) [Without B8] = 0 | -3.7081 |
0.8534 |
-4.345 |
< 0.001*** |
aSimultaneous tests for general linear hypotheses.
bSignificance values are as follows: p < 0.10; * p < 0.05; ** p < 0.01; and *** p < 0.001. Adjusted p values reported single-step method.
Nighttime Object Detection Accuracy
Table 145 shows the results regarding detecting objects during the nighttime when considering upstream conditions (i.e., this evaluation includes objects located behind distractor signs and after turns). The probability of detecting an object was statistically significantly different for age groups (older drivers were more likely to miss the object). While object type was statistically different during the daytime, it was not during the nighttime. In other words, the probability of missing any of the different objects was similar at night.
Similar to daytime conditions, the upstream condition was not significant. In other words, it did not matter whether the object was beyond a sign with beacons or LEDs or beyond one of the detector signs or not beyond a sign (i.e., following a turn). The probability of missing the object was similar regardless of the upstream condition.
Table 146 shows the results when focusing on the type of beacon/LED of the previous assembly. The base condition is older participant, with the object being a pedestrian located beyond an R-B with a Ped X-ing sign. Similar to the findings in table 145, age group was significant while object type was not. The pair comparison shown in table 147 confirms that the probability of missing an object at night was similar for the three objects. Table 148 confirms the findings that age does affect the probability of missing an object. No statistical difference was identified between the different sign families (Ped X-ing, other common signs, and uncommon signs) as shown in table 149.
Table 150 and table 151are pair comparisons between different beacon/LED types. The only comparisons with a statistical difference involved the sign with the embedded LEDs. Care should be taken with this finding because only one object type was present beyond the LED-embedded signs (the trash can) and the LED-embedded signs were not rotated to other positions on the course because of study limitations.
The comparison between the beacon shape (circular or rectangular) revealed no difference in the probability of missing an object (see table 152). The comparisons regarding beacon placement (above or below the sign) also showed no difference. In other words, the shape and location of the beacon within the sign assembly did not affect the probability of missing an object. Recall that the probability of missing any of the objects at night is higher than during the day.
Table 145. Model for nighttime object detection accuracy considering upstream condition.a
Variables | Value | Std. error | DF | t-value | p-value |
Reference Levelb | -1.19514 | 0.3381 | 732 | -3.535173 | 0.0004 |
O.Y: young | -1.20987 | 0.4271 | 34 | -2.83279 | 0.0077 |
Object: box | 0.269929 | 0.2202 | 732 | 1.22575 | 0.2207 |
Object: can | 0.086253 | 0.2247 | 732 | 0.383848 | 0.7012 |
Upstream2: Distractor | 0.178722 | 0.1867 | 732 | 0.957376 | 0.3387 |
Upstream2: Turn | -0.40148 | 0.2404 | 732 | -1.669795 | 0.0954 |
aNotes:
- 1. Linear mixed-effects model fit by maximum likelihood.
- 2. Fixed effects: Miss ~ O.Y + Object + Upstream2.
- O.Y. = older driver.
- Object = pedestrian.
- Upstream2 = assembly.
Table 146. Model for nighttime object detection accuracy considering upstream assembly.a
Variables | Value | Std. error | DF | t-value | p-value |
Reference Levelb | -2.04057 | 0.53165 | 269 | -3.83818 | 0.0002 |
O.Y: young | -1.14365 | 0.460748 | 34 | -2.48216 | 0.0182 |
Object: can | 0.547958 | 0.417624 | 269 | 1.312085 | 0.1906 |
Object: ped | 0.396126 | 0.334421 | 269 | 1.184514 | 0.2373 |
Prev.Assembly: C-A12 | -0.063 | 0.539061 | 269 | -0.11686 | 0.9071 |
Prev.Assembly: C-B12 | 0.402231 | 0.477727 | 269 | 0.841968 | 0.4006 |
Prev.Assembly: C-B8 | 0.212159 | 0.58904 | 269 | 0.360178 | 0.7190 |
Prev.Assembly: C-V12 | 0.499291 | 0.569511 | 269 | 0.876701 | 0.3814 |
Prev.Assembly: R-A | 0.955322 | 0.469532 | 269 | 2.034627 | 0.0429 |
Prev.Assembly: LEDs | 1.888127 | 0.554063 | 269 | 3.407785 | 0.0008 |
Sign.Fam: Other Common | -0.51713 | 0.639166 | 269 | -0.80907 | 0.4192 |
Sign.Fam: Uncommon | 0.558717 | 0.403549 | 269 | 1.384508 | 0.1674 |
aNotes:
- 1. Linear mixed-effects model fit by maximum likelihood.
- 2. Fixed effects: Miss ~ O.Y + Object + Prev.Assembly + Sign.Fam
- O.Y. = older driver.
- Object = pedestrian.
- Prev.Assembly = R-B.
- Sign.Fam = common.
Table 147. Nighttime object detection accuracy, multiple comparisons by object type.
Linear hypothesesa | Estimate | Std. error | z-value | Pr(>|z|) |
Box - Ped = 0 | -0.3961 | 0.3287 | -1.205 | 0.447 |
Can - Ped = 0 | 0.1518 | 0.3724 | 0.408 | 0.912 |
Can - Box = 0 | 0.548 | 0.4104 | 1.335 | 0.373 |
aSimultaneous tests for general linear hypotheses.
Table 148. Nighttime object detection accuracy, multiple comparisons by age group.
Linear hypothesesa | Estimate | Std. error | z-value | Pr(>|z|) b |
Young - Old = 0 | -1.1437 | 0.4528 | -2.526 | 0.0115* |
aSimultaneous tests for general linear hypotheses.
bSignificance values are as follows: p < 0.10; * p < 0.05; ** p < 0.01; and *** p < 0.001. Adjusted p values reported—single-step method.
Table 149. Nighttime object detection accuracy, multiple comparisons by sign family.
Linear hypothesesa | Estimate | Std. error | z-value | Pr(>|z|) |
(Ped X-ing) - (Other Common) = 0 | 0.5171 | 0.6281 | 0.823 | 0.671 |
(Ped X-ing) - (Uncommon) = 0 | -0.5587 | 0.3966 | -1.409 | 0.317 |
(Uncommon) - (Other Common) = 0 | 1.0758 | 0.7619 | 1.412 | 0.315 |
aSimultaneous tests for general linear hypotheses.
Table 150. Nighttime object detection accuracy, multiple comparisons to reference assembly by assembly type.
Linear hypothesesa | Estimate | Std. error | z-value | Pr(>|z|) b |
(C-A12) - ( R-B ) = 0 | -0.063 | 0.5298 | -0.119 | 1.0000 |
(C-B12) - ( R-B ) = 0 | 0.4022 | 0.4695 | 0.857 | 0.9132 |
(C-B8 ) - ( R-B ) = 0 | 0.2122 | 0.5789 | 0.366 | 0.99877 |
(C-V12) - ( R-B ) = 0 | 0.4993 | 0.5597 | 0.892 | 0.89772 |
( R-A ) - ( R-B ) = 0 | 0.9553 | 0.4614 | 2.07 | 0.17552 |
(LEDs) - ( R-B ) = 0 | 1.8881 | 0.5445 | 3.468 | 0.00298** |
aSimultaneous tests for general linear hypotheses.
bSignificance values are as follows: p < 0.10; * p < 0.05; ** p < 0.01; and *** p < 0.001. Adjusted p values reported single-step method.
Table 151. Nighttime object detection accuracy, other multiple comparisons by assembly type.
Linear hypothesesa | Estimate | Std. error | z-value | Pr(>|z|) b |
(C-B12) - (C-A12) = 0 | 0.46523 | 0.47666 | 0.976 | 0.9225 |
(C-B8 ) - (C-A12) = 0 | 0.27515 | 0.6768 | 0.407 | 0.9985 |
(C-V12) - (C-A12) = 0 | 0.56229 | 0.66282 | 0.848 | 0.9564 |
(R-A) - (C-A12) = 0 | 1.01832 | 0.4899 | 2.079 | 0.2904 |
(LEDs) - (C-A12) = 0 | 1.95112 | 0.59876 | 3.259 | 0.0136* |
(C-B8 ) - (C-B12) = 0 | -0.19007 | 0.6011 | -0.316 | 0.9996 |
(C-V12) - (C-B12) = 0 | 0.09706 | 0.57848 | 0.168 | 1.0000 |
(R-A) - (C-B12) = 0 | 0.55309 | 0.44805 | 1.234 | 0.8137 |
(LEDs) - (C-B12) = 0 | 1.4859 | 0.54986 | 2.702 | 0.0721 |
(C-V12) - (C-B8) = 0 | 0.28713 | 0.5532 | 0.519 | 0.9952 |
(R-A) - (C-B8) = 0 | 0.74316 | 0.60602 | 1.226 | 0.8179 |
(LEDs) - (C-B8) = 0 | 1.67597 | 0.60419 | 2.774 | 0.0595 |
(R-A) - (C-V12) = 0 | 0.45603 | 0.56416 | 0.808 | 0.9645 |
(LEDs) - (C-V12) = 0 | 1.38884 | 0.61456 | 2.26 | 0.2045 |
(LEDs) - (R-A) = 0 | 0.93281 | 0.54366 | 1.716 | 0.5117 |
aSimultaneous tests for general linear hypotheses.
bSignificance values are as follows: p < 0.10; * p < 0.05; ** p < 0.01; and *** p < 0.001. Adjusted p values reported single-step method.
Table 152. Nighttime object detection accuracy for beacon placement.
Linear hypothesesa |
Estimate |
Std. error |
z-value |
Pr(>|z|) |
(Circular) - (Rectangular) = 0 |
-0.2939 |
0.3233 |
-0.909 |
0.657 |
(Above) - (Below)[All] = 0 |
0.2414 |
0.3595 |
0.671 |
0.810 |
(Above) - (Below)[Without B8] = 0 |
0.2450 |
0.3395 |
0.722 |
0.779 |
aSimultaneous tests for general linear hypotheses.
Speed When Object Was Detected or Missed
During reviews of the previous finding, a question was asked on whether the drivers who missed the pedestrians and the trash cans were driving faster compared with the drivers who detected the objects. The speed when the participant detected the object was readily available. The speed when a participant missed the object was not as readily available. The research team searched the data stream to identify the speed when the driver who missed an object was near the location of the missed object. Table 153 provides the average speeds. When speed was included in the statistical evaluation, it was found to be not significant. The drivers who missed the pedestrian or the trash can during the study were operating the vehicle at similar speeds as the drivers who detected the pedestrian or trash can, both in the daytime and the nighttime.
Table 153. Average speed of vehicle when object was detected or missed.
Day or night | Object | Object missed | Object detected | ||||
Average speed (mi/h) |
Standard deviation of speed (mi/h) |
Count |
Average speed (mi/h) |
Standard deviation of speed (mi/h) |
Count | ||
Day | Can | 35 | 5.4 | 19 | 33 | 8.2 | 263 |
Day | Ped | 37 | 3.1 | 2 | 38 | 5.9 | 210 |
Day | Either | 36 | 5.2 | 21 | 35 | 7.7 | 473 |
Night | Can | 32 | 6.4 | 58 | 33 | 5.7 | 227 |
Night | Ped | 35 | 4.3 | 44 | 34 | 6.4 | 167 |
Night | Either | 34 | 5.8 | 102 | 34 | 6.0 | 394 |
Key Findings Regarding Object Detection Accuracy
For the analysis focusing on the accuracy of detecting objects, which considered the number of objects missed by the participants, results indicate the following:
- As expected, there is a significant difference in the probability of missing objects between daytime and nighttime conditions. What was not expected was the magnitude of the difference. Overall, during the day, 1 in 23 pedestrians/trash cans were missed while at night 1 in 5 pedestrians/trash cans were missed.
- For both daytime and nighttime conditions, the shape of the beacon did not matter; a similar probability of missing the object was present whether the beacons were circular or rectangular.
- The drivers who missed the pedestrian or the trash can during the study were operating the vehicle at speeds similar to the drivers who detected the pedestrian or trash can, both in the daytime and the nighttime. For this study, the speed the participant was driving did not affect whether an object was missed.
- The location of the beacons (above or below the sign) was significant during the day but not at night. During the day, participants were less likely to miss an object when the beacons were above the sign.
Raw data for the discomfort glare study are shown in figure 64 for C-B8 at position 1, figure 65 for C-B8 at position 2, figure 66 for LED at position 1, and figure 67 for LED at position 2. The abbreviations for these figures include the following:
- P1 = position 1 or 250 ft in advance of assembly.
- P2 = position 2 or 150 ft in advance of assembly.
- C = Comfortable—the glare is not annoying and the signal is easy to look at.
- I = Irritating—the glare is uncomfortable; however, the participant is still able to look at it without the urge to look away.
- U = Unbearable—the glare is so intense that the participant wants to avoid looking at it.
The data show that for all devices at all distances, the percentage of participants indicating the brightness of the lights from the beacons/LEDS was comfortable decreased as brightness increased and the percentage of participants indicating the discomfort glare was unbearable increased as brightness increased. In addition, the data show that almost 50 percent of all participants indicated the discomfort glare was unbearable at a setting number 6 for all devices.
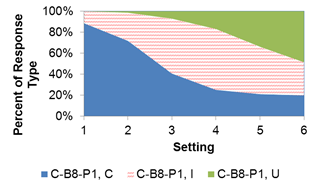
Figure 64. Graph. Percent of response for discomfort glare study—C-B8 at position 1.
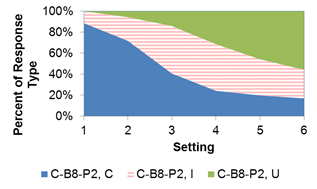
Figure 65. Graph. Percent of response for discomfort glare study—C-B8 at position 2.
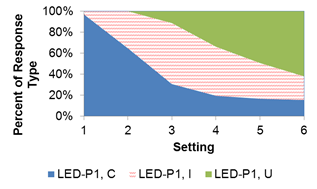
Figure 66. Graph. Percent of response for discomfort glare study—LED at position 1.
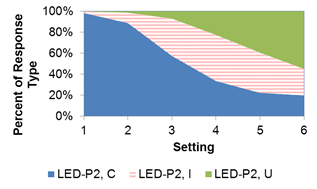
Figure 67. Graph. Percent of response for discomfort glare study—LED at position 2.
The statistics program R was used to develop cumulative logistic regression models to evaluate participant’s ratings of discomfort glare. Cumulative logistic regression models are a method for calculating the probability of a response less than or equal to a specific value for ordinal data; the discomfort ratings in this study (comfortable, irritating, and unbearable) are ordinal data. The basic form of the Cumulative Logit models in this study is shown in figure 68 (32):

Figure 68. Equation. Basic form of the Cumulative Logit model.
Where:
Logit(p) = odds(p) = ln(p) – ln(1-p).
P = P(I_ciu ≤ j).
I_ciu = discomfort level, such that I_ciu ∈{1,2,3} → 〈comfortable, irritating, unbearable〉
Ln = the natural log function.
P(I_ciu ≤ j) = probability of I_ciu not exceeding threshold j, such that j ∈ {1,2,3}.
αj = intercept value (for comfortable j = 1, for irritating j = 2).
βi = ith parameter value.
Xi = ith variable.
To calculate the probability of event I_ciu = 3 (unbearable brightness), the equation in figure 69 is valid.

Figure 69. Equation. Probability of unbearable brightness.
Where:
P(I_ciu =3) = probability of I_ciu equal 3 (unbearable).
P(I_ciu ≤ 2) = probability of I_ciu ≤ 2 (comfortable or irritating).
α2 = intercept value for P(I_ciu ≤ 2).
βi = ith parameter value.
Xi = ith variable value.
Exp = the exponential function.
For variables only taking two values, exp (βi) is the odds ratio, which is the probability of eventA occurring divided by the probability of event B occurring. Mathematically, the odds ratio is as shown in figure 70:

Figure 70. Equation. Basic form of the odds ratio for events A and B.
Where:
Pa = probability of event A occurring.
Pb = probability of event B occurring.
The variables considered for inclusion in the statistical models are defined in table 154. To select statistically significant cumulative logistic regression models, researchers used the Akaike’s Information Criterion (AIC) to assess the value of adding a parameter to the model. First, researchers tested the three measures of brightness (C_Level, INT_One, and OP_One) to determine which parameter had the lowest AIC. Next, researchers tested the non-brightness measures for inclusion in the model, adding one parameter at a time, adding the parameter with the lowest AIC. Models with lower AIC values fit the data better than models with higher AIC values while limiting the number of parameters in the model. To say it another way, the selected models maximize log-likelihood while minimizing the number of parameters in the model. Researchers did not investigate the inclusion of cross terms in this study.
Table 154. Discomfort glare variable names and descriptions.
Variable name |
Description |
I_ciu | Indicates whether the participant felt the device was comfortable, irritating, or unbearable to look at for a given combination of variables; equals 1 if comfortable, 2 if irritating, and 3 if unbearable. |
INT_One | 95th percentile intensity measured at a vertical angle of zero and a horizontal angle of zero. |
OP_One | Optical power measured at a vertical angle of zero and a horizontal angle of zero. |
C_Level | Setting on the controller for a given observation; equals 1 through 6 as categorical variables. |
M_Num | Measurement number representing the order measurements were taken (0 = 250 ft for sign 1, 1 = 150 ft for sign 1, 2 = 250 ft for sign 2, 3 = 250 ft for sign 2) |
I_Age | Indicator value for age of participant; equals 1 for participants 55 years or older, 0 otherwise. |
I_Dist | Indicator value for distance from assembly where measurements were taken; 1 if 250 ft, 0otherwise. |
I_Device | Indicator value for device where measurements were taken; 1 if sign 1, 0 if sign 2. |
I_Day | Indicator value for day or night; 1 if day, 0 if night. |
After selecting an appropriate cumulative logistic regression model with C_Level as the measure of brightness, researchers generated two additional models using OP_One and INT_One as the parameters representing the brightness of the device; these models allow researchers to compare participants’ rating of discomfort glare with measures of brightness found in SAE standard J595. The models evaluated as part of this process and associated AIC values are shown in table 155. Note that the only difference between the three selected models is the parameter used to represent the brightness of the device.
Table 155. Model specification and selection using Akaike Information Criterion (AIC).
Modela |
Number of parameters |
Log- likelihood |
AIC |
Model selected for further analysis |
INT_One | 3 |
-1,696 |
3,398 |
No |
OP_One | 3 |
-1,563 |
3,131 |
No |
C_Level | 7 |
-1,421 |
2,856 |
Yes |
C_Level+M_Num | 10 |
-1,409 |
2,838 |
No |
C_Level+I_Age | 8 |
-1,418 |
2,846 |
No |
C_Level+I_Dist | 8 |
-1,415 |
2,854 |
No |
C_Level+I_Device | 8 |
-1,421 |
2,857 |
No |
C_Level+I_Day | 8 |
-1,173 |
2,363 |
Yes |
C_Level+I_Day+M_Num | 11 |
-1,158 |
2,339 |
Yes |
C_Level+I_Day+I_Age | 9 |
-1,169 |
2,356 |
No |
C_Level+I_Day+I_Dist | 9 |
-1,172 |
2,361 |
No |
C_Level+I_Day+I_Device | 9 |
-1,173 |
2,364 |
No |
C_Level+I_Day+M_Num+I_Age | 12 |
-1,154 |
2,332 |
Yes |
Selected Models | ||||
C_Level+I_Day+M_Num+I_Age | 12 |
-1,154 |
2,332 |
Yes |
OP_One+I_Day+M_Num+I_Age | 8 |
-1169 |
2,352 |
Yes |
INT_One+I_Day+M_Num+I_Age | 8 |
-1216 |
2,448 |
Yes |
aModels including M_Num are not full rank (cannot be evaluated) with either I_Dist or I_Device.
Selected Cumulative Logit Regression Models
The parameter estimates, standard error, odds ratio, and 95-percent confidence interval for the odds ratio for the selected Cumulative Logit Regression Models are provided in table 156 through table 158. The models show that as brightness increases, the odds of a participant indicating the brightness is less than or equal to a rating of irritating decreases, which means that as brightness increases the odds of a participant indicating the discomfort glare is unbearable increases. In addition, the models show the participant’s odds of indicating unbearable discomfort is about 13 times greater at night than during the day. An atypical finding in this study is that the odds of a younger participant indicating unbearable discomfort are about 1.4times more likely than older participant’s odds of indicating unbearable discomfort. In the next section, researchers use the models in table 157 and table 158 to develop equations predicting the probability of a participant indicating a light source has unbearable discomfort.
Table 156. Cumulative logit model with C_Level as a measure of brightness.
Parameter | Estimate | Standard | 95-percent confidence intervala | ||
Lower bounds |
Odds ratiob |
Upper bounds | |||
Intercept (j = 1) | 1.5207 |
0.2655 |
NA |
NA |
NA |
Intercept (j = 2) | 4.1643 |
0.2808 |
NA |
NA |
NA |
C_Level = 2 | -1.7627 |
0.2897 |
0.0972 |
0.1716 |
0.3027 |
C_Level = 3 | -3.4831 |
0.2844 |
0.0176 |
0.0307 |
0.0536 |
C_Level = 4 | -4.6302 |
0.2878 |
0.0055 |
0.0098 |
0.0171 |
C_Level = 5 | -5.3249 |
0.2938 |
0.0027 |
0.0049 |
0.0087 |
C_Level = 6 | -5.9668 |
0.3036 |
0.0014 |
0.0026 |
0.0046 |
I_Day = 1 | 2.6209 |
0.1319 |
10.6161 |
13.7481 |
17.8040 |
M_Num = 2 | 0.7791 |
0.1580 |
1.5991 |
2.1796 |
2.9708 |
M_Num = 3 | 0.4887 |
0.1563 |
1.2000 |
1.6302 |
2.2145 |
M_Num = 4 | 0.1285 |
0.1552 |
0.8389 |
1.1371 |
1.5414 |
I_Age = 1 | 0.3302 |
0.1109 |
1.1194 |
1.3912 |
1.7290 |
aIf the odds ratio confidence interval includes the value 1.000 (which M_Num = 4 does), the difference is not statistically significant with 95-percent confidence.
bFor C_Level = z, the odds ratio is odds(C_Level = z)/odds(C_Level = 1). For M_Num = z, the odds ratio is odds(M_Num = z)/odds(M_Num = 1).
NA = Not Applicable.
Table 157. Cumulative logit model with OP_One as a measure of brightness.
Parameter |
Estimate |
Standard |
95-percent confidence intervala |
||
Lower bounds |
Odds ratiob |
Upper bounds |
|||
Intercept (j = 1) | 4.0117 |
0.2602 |
NA |
NA |
NA |
Intercept (j = 2) | 6.6042 |
0.2984 |
NA |
NA |
NA |
OP_Onec | -0.0001534 |
0.000006193 |
NA |
NA |
NA |
I_Day = 1 | 2.5577 |
0.1287 |
10.0287 |
16.6091 |
12.9061 |
M_Num = 2 | 0.8730 |
0.1661 |
1.7288 |
3.3153 |
2.3941 |
M_Num = 3 | -2.1270 |
0.1875 |
0.0825 |
0.1721 |
0.1192 |
M_Num = 4 | -2.4520 |
0.1903 |
0.0593 |
0.1251 |
0.0861 |
I_Age = 1 | 0.3313 |
0.1105 |
1.1216 |
1.7296 |
1.3928 |
aIf the odds ratio confidence interval includes the value 1.000, the difference is not statistically significant with 95-percent confidence.
bFor M_Num = z, the odds ratio is odds(M_Num = z)/odds(M_Num = 1).
cIt is not appropriate to interpret the parameter estimate of OP_One as an odds ratio because it is a continuous variable.
NA = Not Applicable.
Table 158. Cumulative logit model with INT_One as a measure of brightness.
Parameter | Estimate | Standard | 95-percent confidence intervala | ||
Lower bounds |
Odds ratiob |
Upper bounds | |||
Intercept (j = 1) | 5.5347 |
0.3490 |
NA |
NA |
NA |
Intercept (j = 2) | 7.9764 |
0.3819 |
NA |
NA |
NA |
INT_Onec | -0.003781 |
0.0001693 |
NA |
NA |
NA |
I_Day = 1 | 2.4113 |
0.1238 |
8.7465 |
11.1484 |
14.2100 |
M_Num = 2 | 0.8889 |
0.1689 |
1.7469 |
2.4325 |
3.3870 |
M_Num = 3 | -4.7285 |
0.2840 |
0.0051 |
0.0088 |
0.0154 |
M_Num = 4 | -5.0237 |
0.2871 |
0.0037 |
0.0066 |
0.0116 |
I_Age = 1 | 0.3205 |
0.1085 |
1.1139 |
1.3778 |
1.7043 |
aIf the odds ratio confidence interval includes the value 1.000, the difference is not statistically significant with 95-percent confidence.
bFor M_Num = z, the odds ratio is odds(M_Num = z)/odds(M_Num = 1).
cIt is not appropriate to interpret the parameter estimate of INT_One as an odds ratio because it is a continuous variable.
NA = Not Applicable.
To assist in evaluating the relationship between unbearable discomfort glare, optical power, and 95th percentile peak intensity, researchers developed prediction equations. These equations predict the probability of a participant indicating beacons in this study had unbearable discomfort glare. The prediction equations are shown in figure 71 and figure 72.

Figure 71. Equation. Probability of unbearable discomfort glare based on optical power.

Figure 72. Equation. Probability of unbearable discomfort glare based on intensity.
Where:
p(I_ciu = 3)OP = Probability of participant indicating the light level is unbearable with optical power as the measure of brightness.
p(I_ciu = 3)INT = Probability of participant indicating the light level is unbearable with 95th percentile intensity as the measure of brightness.
exp( ) = Exponential function.
OP_one = Optical power of the device measured at a vertical and horizontal angle of zero.
INT_one = 95th percentile intensity of the device measured at a vertical and horizontal angle of zero.
I_Day = Indicator variable for daytime; equals 1 if daytime and 0 if nighttime.
I_Age = Indicator variable for age group; equals 1 if 55 years or older, 0 otherwise.
fM_Num(OP) = Factor associated with the measurement number (M_Num); equals 0 if M_Num = 1, 0.0.8730 if M_Num = 2, -2.1270 if M_Num = 3, and -2.4520 if M_Num = 4.
fM_Num(INT) = Factor associated with the measurement number (M_Num); equals 0 if M_Num = 1, 0.8889 if M_Num = 2, -4.7285 if M_Num = 3, and -5.0237 if M_Num = 4.
Using these prediction equations, researchers produced the curves shown in figure 73 and
figure 74; these curves show the probability of an older driver (55 years or more) indicating the discomfort glare of LED embedded sign is unbearable using the flash pattern from the right beacon of an RRFB for daytime and nighttime conditions. In addition to the discomfort glare curves, the graphs also indicate the SAE minimum for test point and three times the SAE minimum for the test point. Specific values for the optical power and 95th percentile intensity when 10 percent, 15 percent, and 50 percent of the older driver population would indicate the discomfort is unbearable are provided in table 159; in addition, the percentiles at the SAE minimum and three times the SAE minimum are shown in table 160.
Key Findings for Discomfort Glare Study
The data show that for all devices at all distances, the percentage of participants indicating the brightness of the lights from the beacons/LEDs is comfortable decreases as brightness increases, and the percentage of participants indicating the discomfort glare is unbearable increases as brightness increases.
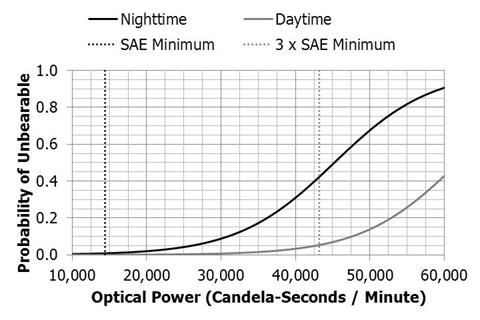
Figure 73. Graph. Older drivers’ probability of unbearable discomfort glare by optical power and time of day for LED-embedded signs at 250 ft.
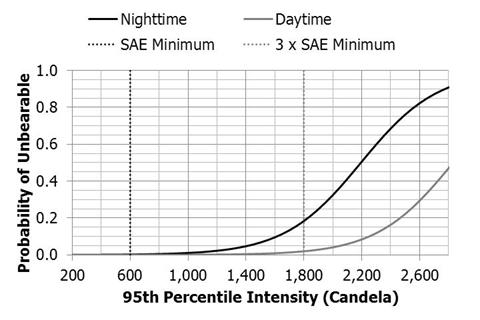
Figure 74. Graph. Older drivers’ probability of unbearable discomfort glare by 95th percentile intensity and time of day for LED-embedded signs at 250 ft.
Table 159. Unbearable discomfort glare for 10th, 15th, and 50th percentiles for LED-embedded signs at 250 ft.
Brightness variable |
I_Day |
10th percentile |
15th percentile |
50th percentile |
SAE minimuma |
3 times SAE minimumb |
OP_One |
Day |
48,190 |
51,246 |
62,703 |
14,400 |
43,200 |
Night |
31,296 |
34,352 |
45,809 |
14,400 |
43,200 | |
INT_One |
Day |
02,251 |
02,373 |
02,832 |
00,600 |
01,800 |
Night |
01,613 |
01,736 |
02,194 |
00,600 |
01,800 |
aThis is the minimum measured at a vertical angle of zero and horizontal angle of zero.
bThis is a potential maximum brightness; the Institute of Transportation Engineers specifies a maximum peak luminous intensity on traffic signals of three times the minimum intensity value.
Table 160. Unbearable discomfort glare percentiles at SAE minimum and three times the SAE minimum for LED-embedded signs at 250 ft.
Brightness variable |
I_Day |
SAE minimuma |
Probability unbearable (percent) |
3 times SAE minimumb |
Probability unbearable (percent) |
OP_One |
Day |
14,400 |
0.07 |
43,200 |
04.96 |
Night |
14,400 |
0.85 |
43,200 |
40.25 | |
INT_One |
Day |
00,600 |
0.02 |
01,800 |
01.98 |
Night |
00,600 |
0.24 |
01,800 |
18.38 |
aThis is the minimum measured at a vertical angle of zero and horizontal angle of zero.
bThis is a potential maximum brightness; the Institute of Transportation Engineers specifies a maximum peak luminous intensity on traffic signals of three times the minimum intensity value.
Observations From Discomfort Glare Study
The data indicate agencies should focus on meeting the SAE minimum intensity and place less emphasis on obtaining the brightest devices possible. When devices have intensities and optical powers close to the SAE minima, the probability of unbearable discomfort glare is less than 1percent.
The profession needs maximum brightness for RRFB beacons. As the brightness of the beacons on a traffic control device increases, the probability of a driver indicating the discomfort glare is unbearable increases. When the discomfort glare is unbearable, drivers are more likely to divert their eyes away from the discomfort, which may result in drivers missing hazards located near the glare source.
When setting maximum brightness for RRFB beacons, decision makers should consider a variety of distances from the glare source. As the distance from the glare source varies, drivers perceive increases and decreases in discomfort glare. A policy on maximum brightness should limit the discomfort glare along the length of the approach with a focus on critical distances such a decision sight distance, stopping sight distance, and sign legibility distance.
Setting different nighttime and daytime maximum brightness values might be appropriate. The data indicate that under daytime conditions, participants are 13 times more likely to indicate the brightness is either comfortable or irritating compared with their responses in nighttime conditions (when they were more likely to indicate unbearable instead). This suggests that daytime settings could be higher than nighttime settings.
The discomfort glare results support the concept of dimming traffic control devices during low light conditions; however, this study did not investigate the ability of devices below the SAE minima to draw a driver’s attention, which means dimmed devices should still meet SAE minimum values.
Brightness specifications for RRFBs should consider alternative measures of brightness, such as optical power. Peak intensity is not the only measure of brightness, and in some instances, it may not be the best measure of brightness. When quantifying the brightness of traffic control devices, researchers should consider alternative measures of brightness in their studies and use statistics to determine which measure is the most appropriate.
This study found controller setting (which is highly correlated with intensity and optical power) as the measure of brightness that resulted in the model with the best fit of data. This indicates participants may have been responding to the setting of the controller at measurement locations two, three, and four rather than the brightness of the traffic control device.