Evaluation of The TAPCO Sequential Dynamic Curve Warning System
LITERATURE REVIEW
This section discusses the relationship between roadway geometry, vehicle speeds, and crashes on horizontal curves and reviews the effectiveness of various applications of Dynamic Speed Feedback Sign (DSFS) systems installed to date. This research effort will test the effectiveness of the SDCWS and its impact on safety. Even though several dynamic curve sign systems have been tested in the past, this system is unique in terms of including guidance not just before or at the curve, but also throughout the curve with the blinking chevrons. The results from this research will add to the body of knowledge and provide safety engineers with another tool to address curve crashes.
Relationship between Curve Crash Rate and Geometry
Curves have about three times the crash rate of tangent sections.(1) Preston and Schoenecker reported that 25 to 50 percent of the severe road departure crashes in Minnesota occurred on curves, even though curves account for only 10 percent of the total system mileage. (2) Shankar et al. evaluated divided State highways without median barriers in Washington State and found a relationship between the number of horizontal curves per kilometer and median crossover crashes.(3) Farmer and Lund evaluated single-vehicle fatal and injury rollover crashes using Fatality Analysis Reporting System (FARS) data and data from Florida, Pennsylvania, and Texas.(4) Using logistic regression, they found that the odds of having a rollover on a curved section were 1.42 to 2.15 times the odds of having a rollover on a straight section.
The majority of crashes on curves involve lane departures. A total of 76 percent of curve-related fatal crashes are single vehicles leaving the roadway and striking a fixed object or overturning. Another 11 percent of curve-related crashes are head-on collisions.(5)
The frequency and severity of curve-related crashes have been correlated to a number of geometric factors, including radius, degree of curve, length of curve, type of curve transition, lane and shoulder widths, preceding tangent length, and required speed reduction.
Luediger et al. found that crash rates increase as the degree of curve increases, even when traffic warning devices are used to warn drivers of the curve. (6) Miaou and Lum found that truck crash involvement increases as horizontal curvature increases, depending on the length of curve.(7) Council found that the presence of spirals on horizontal curves reduced crash probability on level terrain but did not find the same effect for hilly or mountainous terrain.(8) Vogt and Bared evaluated two-lane rural road segments in Minnesota and Washington State using Highway Safety Information System (HSIS) data and found a positive correlation between injury crashes and degree of horizontal curve.(9)
Zegeer et al. evaluated curves on two-lane roads in Washington State using a linear regression model.(10) The researchers found that the degree of curve was positively correlated with crashes, while total surface width and presence of spirals were negatively correlated. They also evaluated 10,900 horizontal curves on two-lane roads in Washington State using a weighted linear regression model and found that crash likelihood increases as the degree and length of curve increases.(10) Mohamedshah et al., however, found a negative correlation between crashes and degree of curve for two-lane roadways.(11)
Preston and Schoenecker examined severe roadway departure crashes and found that 90 percent of fatal crashes and 75 percent of injury crashes occurred on curves with a radius of less than 1,500 ft. (2) Milton and Mannering evaluated 2,725 miles of highway in Washington State using a negative binomial model and reported that an increase in radius was associated with decreases in crash frequency.(12) They also found that a shorter tangent length between horizontal curves was associated with decreases in crash frequency. They speculated that drivers may be traveling at lower speeds and are therefore more likely to be paying attention when tangent lengths between curves are short.
In contrast, Deng et al. evaluated head-on crashes on 729 segments of two-lane roads in Connecticut using an ordered probit model. (13) They included geometric characteristics in the analysis but did not find that the presence of horizontal or vertical curves was significant.
Taylor et al. evaluated the relationship between speed and crashes on rural single-carriageway roads in England. (14) The authors collected data from 174 road sections with 60 mph speed limits with a wide range of conditions. Data collected included injury crash data, traffic volume, speed data, and roadway geometry. Speed and flow were measured at each site for 1 or 2 days, and various speed metrics were calculated, including mean speed, 85th percentile speed, and standard deviation of speed. The authors found that crashes were more highly correlated with mean speed than any other speed metric. They also found that crash frequency increased with mean speed. In general, a 10 percent increase in mean speed resulted in a 26 percent increase in the frequency of injury crashes.
More recently, Khan et al. analyzed curves in Wisconsin to determine the relationship between safety, horizontal curve signs, and geometry. (15) Compared to previous research, a larger data set with greater detail was used to develop a model showing the relationship of the horizontal curves. The data showed that crashes increased with an increase in annual average daily traffic (AADT), posted speed, and curve length; they also increased with a decrease in curve radius. In addition, an analysis of traffic control signs indicated that sites with curve signs (W1-2) had fewer crashes than sites with turn signs (W1-1). Sharper curves, however, showed no significant correlation to sign type in reducing crashes because of other, more substantial influencing factors.
Relationship between Curve Crash Rate and Speed of Curve Negotiation
Although curve-related crashes are correlated to geometric factors, driver factors such as speed selection also contribute to curve-crash frequency and outcome. Driver factors include driver workload, driver expectancy, and speed selection.
Speeding, defined by FHWA as "exceeding the posted speed limit or driving too fast for conditions," is generally problematic. Council et al. evaluated FARS, General Estimates System (GES), and HSIS data to assess the impact of speeding on fatal crashes. (16) Using 2005 FARS data, they found that 29.5 percent of fatal crashes were speed-related. They conducted several different types of analyses and found the single-vehicle run-off-road crashes are more likely to be speed-related than are multi-vehicle crashes. Crashes on curves were more likely to be speed-related as compared to tangent sections and nighttime crashes. Additionally, FARS data indicated that 54 percent of speed-related rollover/overturn, jackknife, or fixed object crashes were on curves. (16)
FHWA estimates that approximately 56 percent of run-off-road fatal crashes on curves are speed-related. (6) The vehicle speed reduction from the tangent section required for traversing a curve has an impact on the frequency and severity of crashes in curves. Abrupt changes in operating speed resulting from changes in horizontal alignment are suggested to be a major cause of crashes on rural two-lane roadways.(6)
Anderson and Krammes developed a model comparing mean speed reduction and mean crash rate for 1,126 horizontal curves on rural two-lane roadways. (17) They reported that the relationship between mean crash rate and required speed reduction to negotiate the curve is roughly linear. This finding is also supported by Fink and Krammes, who indicated that curves requiring no speed reduction did not have significantly different mean crash rates than their preceding roadway tangent.(18)
Driver errors on horizontal curves are often due to the inappropriate selection of speed and the inability to maintain lane position. Drivers' speed selection at curves depends on both explicit attentional cues and implicit perceptual cues. (19) A driver's speed prior to entering a curve has a significant effect on his or her ability to negotiate the curve successfully. (2) Inappropriate speed selection and lane positioning can be a result of a driver failing to notice an upcoming curve or misperceiving the roadway curvature.
Driver workload plays an important role in driver speed maintenance. Distracting tasks such as radio-tuning or cell phone conversations can draw a driver's attention away from speed monitoring, detection of headway changes, lane keeping, and detection of potential hazards.(19) Other factors include sight distance issues, fatigue, or complexity of the driving situation.(19,20)
Preston and Shoenecker evaluated vehicle paths through a curve on a two-lane rural roadway as part of an evaluation of a dynamic curve message sign.(2) The roadway had a posted speed limit of 55 mph and AADT of 3,250 vehicles per day (vpd). The researchers collected data over a 4-day period and randomly selected and evaluated 589 vehicles. A total of 340 of the vehicles (58 percent) were traveling over 55 mph, and the rest were traveling at or below the speed limit. The authors evaluated whether each vehicle successfully negotiated the curve. Vehicles that crossed a left or right lane line on one or more occasions were defined as "not successfully navigating the curve."
A logistic regression model was developed to determine the relationship between initial speed and the probability of a vehicle unsuccessfully navigating the curve. The researchers found that there was a 20 percent better chance for vehicles that were traveling at or below the speed limit to successfully navigate the curve than for vehicles that were traveling over the speed limit, with the difference being statistically significant at 99 percent. They found that 45 percent of vehicles traveling at or above 65 mph were unable to negotiate the curve compared to 30 percent for vehicles that were traveling under 65 mph, with the difference being statistically significant at the 90 percent confidence interval.
Hassan and Easa found that driver misperception of curvature was greatest when vertical curvature was combined with horizontal curvature. (21) This was particularly a problem when a crest vertical curve was superimposed on a severe horizontal curve, or when a sag vertical curve was combined with a horizontal curve, causing the horizontal curve to appear less severe and resulting in drivers underestimating the curve.
Charlton conducted a simulator study and evaluated driver speed adjustments on several types of curves with several types of signing.(20) Charlton found that, in general, drivers approached and entered curves at higher speeds when engaged in cell phone tasks than when in non-distraction scenarios.
Effectiveness of DSFS Systems
DSFS systems have been used in only a few cases to reduce speeds and warn drivers of upcoming curves. They have been used more extensively for a number of other related applications. A summary of information about the application of DSFS on curves and in related situations is provided below.
Bertini et al. studied the effectiveness of a DSFS system on Interstate 5 near Myrtle Creek, Oregon. (22) The system consisted of two displays that provided different messages to drivers based on the speed detected, as shown in table 1.
Sign Panel | Sign Messages | ||
---|---|---|---|
Detected Vehicle Speeds Less than 50 mph | Detected Vehicle Speeds 50–70 mph | Detected Vehicle Speeds over 70 mph | |
1 | CAUTION | SLOW DOWN | SLOW DOWN |
2 | SHARP CURVES AHEAD | YOUR SPEED IS XX MPH |
YOUR SPEED IS OVER 70 MPH |
The curve has an advisory speed of 45 mph with an AADT of 16,750 vpd. Before the DSFS system was in place, there was what the authors termed "dual overhead horizontal alignment/advisory speed combination sign assemblies with 4 flashing beacons." The DSFS system was put in place alongside one of the existing signs in both the northbound and southbound directions. Each system consisted of the actual dynamic message sign, a radar unit, a controller unit, and computer software. Figures 1 and 2 show the system.
The researchers collected speed data using a laser gun. Results indicated that, after installation of the DSFS system, passenger vehicle speeds were reduced by 2.6 mph and commercial truck speeds were reduced by 1.9 mph, with the results being statistically significant at the 95 percent confidence level. The distribution of speeds shifted to the left after installation of the signs, and the differences were found to be statistically significant based on a 95 percent confidence level using the chi-square test.
Results of a driver survey indicated that 95 percent of drivers surveyed noticed the DSFS system, and 76 percent said they slowed down due to the system.
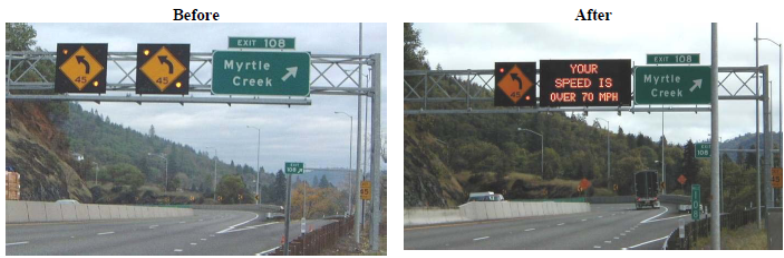
Figure 1. Photo. Northbound Interstate 5 DSFS systems in Oregon.(22)
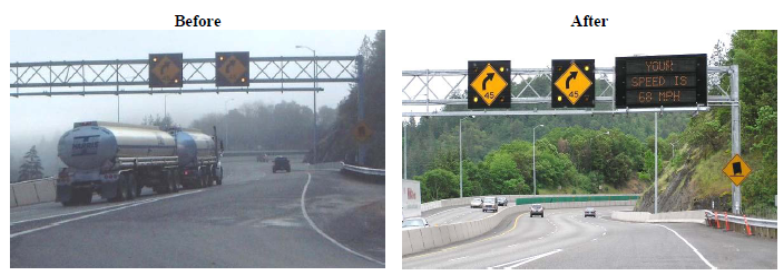
Figure 2. Photo. Southbound Interstate 5 DSFS systems in Oregon.(22)
Another type of DSFS system, a vehicle-activated curve warning sign, was tested on curves in the United Kingdom. (23) Three curve warning signs were placed on two-lane roads in Norfolk, Wiltshire, and West Sussex. The signs, shown in figure 3, were placed 165 to 330 feet before the apex of a curve.
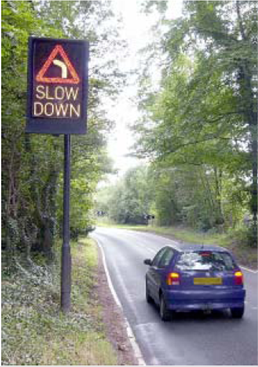
Figure 3. Photo. DSFS system in Norfolk. (23)
The signs were blank when the driver was under a specified speed threshold and displayed the curve sign when a driver exceeded the threshold. The speed threshold was set at the 50th percentile speed for the sign location because the researchers wanted to target the upper half of driver speeds. Once activated, the bend warning display was shown for 4 seconds. Based on previous research, the researchers had calculated this time as being sufficient for drivers to register and understand the message.
Speed data were collected for a minimum of 7 days before the signs were installed, and again 1 month and 1 year after installation. Data were collected at the 1-year period to determine if habituation occurs—in other words, whether drivers become immune to treatments and stop responding. Data were collected using pneumatic tubes at two sites and a radar gun at the third. Mean speeds were reduced by 2.1 mph at West Sussex, 3.0 mph at Wiltshire, and 6.9 mph at Norfolk.
Crash data were available for two sites, and the researchers found that crashes decreased 54 percent at the Norfolk bend site and 100 percent at the Wiltshire bend site. A public survey found that drivers approved of the signs.
The City of Bellevue Washington installed and evaluated 31 DSFS systems, including two used as curve advisory warnings (see figure 4). Both were on urban arterials with 35 mph speed limits and 25 mph advisory speeds. Speeds were collected before and between 18 months and 2 years after installation of the signs. One sign showed a 3.3 mph reduction in 85th percentile speed and the other showed a 3.5 mph reduction.
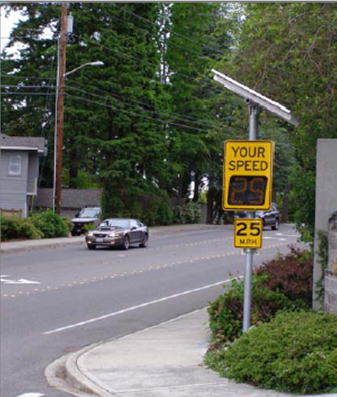
Figure 4 . Photo. DSFS system in Bellevue, Washington.(24)
Preston and Shoenecker also evaluated the safety effect of a DSFS system on County Highway 54 in Minnesota, which is a two-lane rural roadway with a speed limit of 55 mph and an AADT of 3,250 vpd. (2) The curve has an advisory speed of 40 mph. The DSFS system had a changeable message sign and radar unit. A field test was conducted over a 4-day period with a unit that consisted of a closed circuit TV camera, a VCR, and a personal computer. A portable trailer housed the entire system.
The sign displayed the following:
- From 6 a.m. to 10 a.m., 11 a.m. to 2 p.m., and 4 to 7 p.m.: CURVE AHEAD.
- No message during other times of the day unless activated.
During all times of the day, when the radar unit detected a vehicle traveling 53 or more mph, the camera would activate and record the vehicle for 18 seconds. Using a random number generator and depending on the time of day, the computer would either continue displaying the CURVE AHEAD message, display the CURVE AHEAD – REDUCE SPEED message, or display no message.
The team randomly selected 589 of the vehicles captured during data collection and evaluated whether each vehicle successfully negotiated the curve. Successful negotiation was defined as a vehicle remaining within the lane lines as it traversed the curve. Vehicles that crossed a left or right lane line on one or more occasions were defined as "not successfully navigating the curve."
The team found that approximately 35 percent of the drivers who received the message were unable to successfully negotiate the curve. Vehicles that received the CURVE AHEAD sign were more likely to negotiate the curve successfully, but the difference was not statistically significant. Only 26 percent of vehicles that received the CURVE AHEAD – REDUCE SPEED sign were unable to negotiate the curve successfully, and the difference was statistically significant at the 90 percent level of confidence.
Mattox et al. looked at the effectiveness of a DSFS system on secondary highways in South Carolina.(25) This system consisted of a radar device and a 4-ft by 4-ft yellow sign with 6-inch lettering reading YOU ARE SPEEDING IF FLASHING. In addition, there were two 1-ft by 1-ft orange flags and a type B flashing beacon light. Teams collected data in a before-and-after study upstream of the sign, at the sign, and then downstream of the sign. Results showed a significant reduction in speed at the sign and downstream of the sign. Overall mean speed and 85th percentile speeds were reduced by approximately 3 mph.
A report by the California Department of Transportation (Caltrans) provided a summary of the effectiveness of safety treatments in one California district. (26) A changeable message sign was installed at five locations along Interstate 5 to reduce truck collisions. Caltrans reported that truck crashes decreased from 71 percent to 91 percent at four of the sites, while truck crashes increased by 140 percent at one site.
A study by the 3M Company evaluated driver speed feedback signs, which display the approaching drivers' speeds, in the United Kingdom. Signs were tested at various locations in Doncaster, including semi-rural roadways. The sites had speed limits of 40 mph, and reductions up to 7 mph in 85th percentile speeds were noted. (27)
Tribbett et al. evaluated dynamic curve warning systems for advance notification of alignment changes and speed advisories at five sites in the Sacramento River Canyon on Interstate 5. (28) The roadway has high traffic volumes (7,650 to 9,300 vpd), mountainous terrain, and a number of heavy vehicle crashes. The signs were a 10-ft by 7-ft full matrix LED panel that could be programmed to display a variety of messages. Messages used by the researchers included curve warning (shown in figure 5) and driver speed feedback.
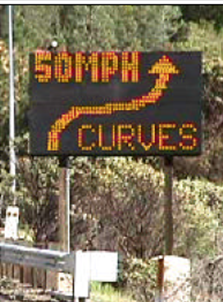
Figure 5 . Photo. Speed warning sign in the Sacramento River Canyon.(26)
The researchers collected speed data using stopwatches. Data were collected before installation of the signs and at several points after the signs were installed; the researchers did not indicate when these after periods were, however. Speed results at the point of curvature (PC) include the following:
- Site 1: statistically significant decreases in mean truck speeds from 2.4 to 5.4 mph and decreases in mean passenger car speeds from 3.0 to 4.5 mph.
- Site 2: no statistically significant changes in truck or passenger car speeds for any time periods.
- Site 3: statistically significant decreases in mean truck speeds from 1.9 to 3.7 mph and increases in passenger cars from 5.2 to 7.8 mph.
- Site 4: no statistically significant change in mean truck speed and a 1.4 mph decrease for passenger cars for one time period that was statistically significant.
- Site 5: a statistically significant change in mean truck speed of 4.5 for one time period and decrease in mean passenger car speeds from 2 to 3 mph.
The researchers also compared 5 years of crash data before installation of the signs and 6 months after. However, due to the very short after period, the results were determined to be unreliable.
The Texas Transportation Institute evaluated the use of a portable speed display trailer in work zones.(29) They found that passenger vehicle speeds were reduced by 7 to 9 mph at one site and 2 to 3 mph at another. Truck speeds were reduced 3 to 10 mph at both sites.
Hallmark et al. also analyzed the installation of DSFS on curves throughout the country to determine the safety benefits.(30) Seven States participated, installing curve warning signs as well as speed feedback signs, which can be seen in figure 6. For the analysis, the mean speed, 85th percentile speed, and the percentage of vehicles going over the speed limit were compared.
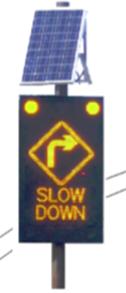
Figure 6 . Photo. Comparison of curve warning sign (left) and speed feedback sign (right). (30)
The average for all of the sites showed a 1.8 mph reduction in mean speed at 1 month, a 2.6 mph reduction in mean speed at 1 year, and a 2.0 mph reduction in speed at 2 years; all of these reductions occurred at the PC. The 85th percentile speed at the PC was reduced by 2.2 mph at both the 1-month and 2-year data collection period and was reduced by 2.9 mph at the 1-year data collection period. Similar decreases were seen in the percentage of vehicles going over the speed limit. The mean speed and 85th percentile speed were also lower at the center of curve, with the largest speed reductions occurring at the 1-month data collection period.
Between the two types of signs, larger decreases were seen with the speed feedback signs than with the curve warning signs. The signs were proven to be effective over time as well. A crash analysis was also performed for the direction of travel for the DSFS and for both directions combined. The analysis showed that, compared to control sites, crashes were reduced 2 to 4 times more for both directions and in the direction of travel by 1.7 to 6.0 times per quarter. Crash modification factors developed using a full Bayes model were .85 for both directions and .97 for the direction of the DSFS.
Sun et al. researched the effectiveness of sequential warning lights as a method to better define the beginning and taper into nighttime work zones. (31) The sequential lights were evaluated in Missouri along Interstate 70 with a right lane closure. Vehicle speeds at the closure were compared in addition to speeds at the point where the vehicle merged and at the lateral position in the taper. Decreases were seen in the mean speed of 2.2 mph and by 1 mph in the 85th percentile speeds, both of which were statistically significant. The lateral position of vehicles in the closed lane increased from 6.2 percent without sequential lights to 7.8 percent with sequential lights. The sequential lights had a negative effect, which could be due to drivers being more aggressive because the taper is illuminated better. The location where vehicles merged was split into eight zones with zone 8 being the zone closest to the taper. With the sequential lights, the total vehicles merging in zones 5 through 7 decreased while the vehicles merging in zones 1 through 4 increased. The exception that occurred was an increase in vehicles merging in zone 8, which further supports the aggressive driver assumption given the lateral position of the driver. Overall, vehicles were merging 20 ft earlier with the sequential lights.
Santiago-Chaparro et al. evaluated the spatial effectiveness of speed feedback signs at a single location along State Highway 164 in Wisconsin. (32) Vehicles were tracked while approaching and receding from the speed feedback signs, and the speeds were monitored to determine when vehicles were slowing down and whether the speed reductions were sustained. The research found that vehicles were reducing their speed the most between 1,200 and 1,400 ft upstream of the speed feedback sign. Speeds began to increase again between 300 to 500 ft downstream of the speed feedback sign, and some vehicles increased speed before even passing the speed feedback sign. The results of the study showed that the speed feedback signs are not adequate for speed reductions at a corridor level but only at the location where the desired speed reduction should occur.
Tracking Vehicles for Data Reduction
Tracking involves monitoring individual vehicles as they traverse multiple data collection points. Limited research has been completed in this area; the literature search revealed only one study that used tracking to reduce data down to only affected vehicles.(33) In this study, tracking was used to determine how much vehicles were slowing down when approaching the sign. This aligns very well with curves, as the speeds of vehicles can be tracked while approaching and through the curve. Vehicles were tracked so that only free-flow passenger vehicles would be analyzed, eliminating vehicles that were influenced by a turning movement. To track the vehicles, the vehicle speed, vehicle length, and time headway were compared at each data collection location.
The standard method was used by determining the mean speed, 85th percentile speed, and percentage of vehicles exceeding the posted speed first in their analysis, but it was not used in determining the effectiveness. Instead, a true effect (TE) was calculated by tracking vehicles and then determining the speed reduction for each vehicle before and after implementation. TE was calculated as shown in figure 7.
True Effect=ΔV 1 - 2, during - ΔV 1 - 2, before
Figure 7 . Equation. True effect.
The tracked data were used to determine the statistics ΔV1-2, during, which is the mean speed reduction between sensors 1 and 2 during the study and ΔV1-2, before, which is the mean speed reduction between sensors 1 and 2 before implementation. Cruzado and Donnell briefly discussed that, depending on the upstream data collection, the data may be over- or underestimated by using only the mean speed reduction at the treatment location. (34) TE better reflects any changes in speeds while approaching the curve.
Another form of tracking was performed by McFadden and Elefteriadou, who determined whether calculating the difference of 85th percentile speeds between two points was significantly different than calculating the 85th percentile of speed reduction between the two points. (33) The 85th percentile of the speed reduction requires that individual vehicles be tracked to determine the speed reduction between the two points. This was achieved using light detection and a light detection and ranging (LIDAR) gun. With the tracked data, the 85th percentile speed reduction was significantly different than the change in 85th percentile speed. The change in the 85th percentile speed underestimated the speed reduction of the vehicles traversing the curve. Hirsh (1987) accounted for this with the differences of the distributions of the two locations.(35)
Misaghi and Hassan expanded on this research in Canada, but instead of using LIDAR guns they used counters. (36) The counters were tracked successfully if three criteria were met at both locations: number of axles, wheel base, and the expected time gap between the two locations. Tolerances were used in the tracking because of variance with the counter clock and inconsistencies with the data collected. Once complete, the speed reduction could be calculated between the points and the 85th percentile speed.